Proton-conducting materials are at the forefront of advancing green energy technologies, offering promising solutions for sustainable power generation and storage. These materials, characterized by their ability to efficiently transport protons, are integral to the development of fuel cells, electrolyzers, and other energy conversion devices. By facilitating the movement of protons, they enable the efficient conversion of chemical energy into electrical energy, with water as the only byproduct, thus minimizing environmental impact. The exploration and optimization of proton-conducting materials are crucial for enhancing the performance, durability, and cost-effectiveness of these technologies. As the demand for clean energy solutions intensifies, innovations in proton-conducting materials are paving the way for a more sustainable and energy-efficient future, driving the transition towards a low-carbon economy.
Understanding Proton-Conducting Materials: The Basics and Their Importance in Green Energy
Proton-conducting materials have emerged as a cornerstone in the development of innovative green energy solutions, offering a promising pathway to more efficient and sustainable energy systems. These materials, which facilitate the movement of protons, are integral to the operation of devices such as fuel cells and electrolyzers. Understanding the basics of proton-conducting materials and their significance in the realm of green energy is essential for appreciating their potential impact on future energy technologies.
At the heart of proton-conducting materials is their ability to transport protons, which are positively charged hydrogen ions, through a solid medium. This property is crucial for the operation of proton exchange membrane (PEM) fuel cells, where the movement of protons from the anode to the cathode generates electricity. The efficiency of this process is largely dependent on the proton conductivity of the material used in the membrane. Materials with high proton conductivity enable faster and more efficient energy conversion, which is vital for the performance of fuel cells.
The importance of proton-conducting materials extends beyond their role in fuel cells. They are also pivotal in the development of electrolyzers, which are devices that use electricity to split water into hydrogen and oxygen. This process, known as electrolysis, is a key component in the production of green hydrogen, a clean fuel that can be used in various applications, from powering vehicles to generating electricity. By improving the efficiency of electrolyzers, proton-conducting materials contribute to the broader adoption of hydrogen as a sustainable energy source.
Moreover, the development of advanced proton-conducting materials is closely linked to the pursuit of alternative energy storage solutions. As the world increasingly relies on renewable energy sources like solar and wind, the need for efficient energy storage systems becomes more pressing. Proton-conducting materials can play a role in this context by enabling the creation of more efficient batteries and supercapacitors, which are essential for storing energy generated from intermittent renewable sources.
In addition to their technical applications, proton-conducting materials are also significant from an environmental perspective. By facilitating the development of cleaner energy technologies, these materials help reduce reliance on fossil fuels, thereby decreasing greenhouse gas emissions and mitigating climate change. The shift towards green energy solutions is not only a technological imperative but also an environmental necessity, and proton-conducting materials are at the forefront of this transition.
The ongoing research and development in the field of proton-conducting materials are driven by the need to enhance their performance and reduce costs. Scientists are exploring various materials, including polymers, ceramics, and composites, to identify those with optimal properties for specific applications. Innovations in material science, such as the development of nanostructured materials and hybrid systems, hold promise for further improving the efficiency and durability of proton-conducting materials.
In conclusion, proton-conducting materials are indispensable to the advancement of green energy technologies. Their ability to efficiently transport protons is critical for the operation of fuel cells, electrolyzers, and energy storage systems. As research continues to push the boundaries of what these materials can achieve, their role in shaping a sustainable energy future becomes increasingly significant. By understanding the basics and importance of proton-conducting materials, we can better appreciate their potential to transform the energy landscape and contribute to a cleaner, more sustainable world.
Innovations in Proton-Conducting Polymers for Sustainable Energy Applications
Proton-conducting materials have emerged as a cornerstone in the development of sustainable energy technologies, offering promising solutions to the global energy crisis. These materials, which facilitate the movement of protons, are integral to the operation of devices such as fuel cells and electrolyzers. As the world increasingly shifts towards renewable energy sources, the demand for efficient and cost-effective proton-conducting materials has never been more critical. Recent innovations in proton-conducting polymers have opened new avenues for enhancing the performance and durability of these energy systems, thereby paving the way for more widespread adoption.
One of the most significant advancements in this field is the development of novel polymer electrolytes that exhibit high proton conductivity at low temperatures. Traditional proton-conducting materials, such as Nafion, have been widely used due to their excellent conductivity and mechanical stability. However, they often require high humidity and elevated temperatures to function optimally, which can limit their practical applications. In response to these challenges, researchers have focused on designing new polymer structures that can maintain high conductivity under a broader range of conditions. By incorporating various functional groups and optimizing the polymer backbone, scientists have succeeded in creating materials that not only perform well at lower temperatures but also offer improved chemical and thermal stability.
Moreover, the integration of nanotechnology into proton-conducting polymers has further enhanced their capabilities. By embedding nanoparticles or nanofibers into the polymer matrix, researchers have been able to increase the surface area available for proton transport, thereby boosting conductivity. This approach also allows for the fine-tuning of the material’s mechanical properties, making them more suitable for use in flexible and lightweight energy devices. The synergy between nanotechnology and polymer science has thus led to the creation of hybrid materials that combine the best attributes of both fields, offering a versatile platform for future innovations.
In addition to improving the intrinsic properties of proton-conducting polymers, there is a growing emphasis on sustainability and environmental impact. The synthesis of these materials often involves the use of toxic solvents and energy-intensive processes, which can negate some of the environmental benefits they offer. To address this issue, researchers are exploring green chemistry approaches to develop more sustainable production methods. This includes the use of bio-based monomers and environmentally benign solvents, as well as the implementation of energy-efficient polymerization techniques. By reducing the ecological footprint of proton-conducting materials, these efforts aim to align the production process with the overarching goals of sustainable energy.
Furthermore, the versatility of proton-conducting polymers extends beyond traditional energy applications. They are being explored for use in emerging technologies such as hydrogen sensors, electrochemical actuators, and even in the field of biomedicine for drug delivery systems. This broad applicability underscores the potential of these materials to revolutionize not only the energy sector but also other industries that rely on efficient ion transport.
In conclusion, the innovations in proton-conducting polymers represent a significant leap forward in the quest for sustainable energy solutions. By enhancing the performance, durability, and environmental compatibility of these materials, researchers are laying the groundwork for a new generation of energy technologies that are both efficient and eco-friendly. As the field continues to evolve, it holds the promise of transforming the way we harness and utilize energy, ultimately contributing to a more sustainable future.
The Role of Proton-Conducting Ceramics in Fuel Cell Technology
Proton-conducting ceramics have emerged as a pivotal component in the advancement of fuel cell technology, offering promising pathways for the development of efficient and sustainable energy solutions. As the global demand for clean energy sources intensifies, the role of these materials becomes increasingly significant. Fuel cells, which convert chemical energy into electrical energy through electrochemical reactions, are at the forefront of this energy revolution. Among the various types of fuel cells, proton-conducting ceramics are particularly noteworthy due to their unique properties and potential applications.
Proton-conducting ceramics, often composed of perovskite-type oxides, exhibit high proton conductivity at elevated temperatures. This characteristic makes them ideal candidates for use in solid oxide fuel cells (SOFCs) and protonic ceramic fuel cells (PCFCs). These fuel cells operate at intermediate to high temperatures, typically ranging from 400 to 800 degrees Celsius, which allows for efficient energy conversion and reduced material degradation. The high operating temperatures also facilitate the internal reforming of hydrocarbon fuels, thereby enhancing the overall efficiency of the system.
One of the primary advantages of proton-conducting ceramics in fuel cell technology is their ability to conduct protons rather than electrons. This protonic conduction minimizes the activation energy required for the electrochemical reactions, leading to improved performance and efficiency. Moreover, the use of proton-conducting ceramics can significantly reduce the reliance on expensive and rare materials, such as platinum, which are commonly used as catalysts in other types of fuel cells. This reduction in material costs is crucial for the widespread adoption and commercialization of fuel cell technology.
In addition to their cost-effectiveness, proton-conducting ceramics offer enhanced durability and stability under operating conditions. Their robust nature allows them to withstand the harsh environments typically encountered in fuel cell applications, including high temperatures and corrosive atmospheres. This durability translates into longer lifespans for fuel cells, reducing the need for frequent replacements and maintenance. Consequently, the overall lifecycle costs of fuel cell systems are lowered, making them more attractive for both industrial and residential applications.
Furthermore, the integration of proton-conducting ceramics into fuel cell systems can lead to significant environmental benefits. By facilitating the use of hydrogen as a clean fuel source, these materials contribute to the reduction of greenhouse gas emissions and the mitigation of climate change. Hydrogen, when used in fuel cells, produces only water as a byproduct, making it an environmentally friendly alternative to fossil fuels. The adoption of proton-conducting ceramics in fuel cell technology thus aligns with global efforts to transition towards sustainable energy systems.
Despite the numerous advantages, challenges remain in the development and optimization of proton-conducting ceramics for fuel cell applications. Research is ongoing to enhance their conductivity, reduce operating temperatures, and improve their compatibility with other fuel cell components. Advances in material science and engineering are expected to address these challenges, paving the way for more efficient and cost-effective fuel cell technologies.
In conclusion, proton-conducting ceramics play a crucial role in the evolution of fuel cell technology, offering a viable solution for the generation of clean and sustainable energy. Their unique properties, coupled with ongoing research and development efforts, position them as key enablers in the transition towards a greener energy future. As the world continues to seek innovative solutions to its energy challenges, the importance of proton-conducting ceramics in fuel cell technology is likely to grow, driving further advancements in this promising field.
Advances in Nanostructured Proton-Conducting Materials for Enhanced Performance
Proton-conducting materials have emerged as a cornerstone in the development of advanced energy technologies, particularly in the realm of fuel cells and hydrogen-based systems. These materials, which facilitate the movement of protons through their structure, are critical for the efficient operation of devices that convert chemical energy into electrical energy. Recent advances in nanostructured proton-conducting materials have significantly enhanced their performance, offering promising pathways for the development of innovative green energy solutions.
The unique properties of nanostructured materials, characterized by their high surface area and tunable porosity, have been instrumental in improving proton conductivity. By manipulating the size and shape of these materials at the nanoscale, researchers have been able to optimize pathways for proton transport, thereby increasing the efficiency of energy conversion processes. For instance, the incorporation of nanostructured materials in the design of proton exchange membranes has led to substantial improvements in their conductivity and mechanical stability. This, in turn, has resulted in more durable and efficient fuel cells, which are crucial for the widespread adoption of hydrogen as a clean energy source.
Moreover, the development of composite materials that combine different nanostructures has opened new avenues for enhancing proton conductivity. By integrating materials with complementary properties, researchers have been able to create hybrid systems that exhibit superior performance compared to their individual components. For example, the combination of inorganic nanoparticles with organic polymers has led to the creation of membranes that not only conduct protons more effectively but also exhibit enhanced thermal and chemical stability. This synergy between different materials is a testament to the potential of nanostructured proton-conducting materials in revolutionizing energy technologies.
In addition to improving conductivity, advances in nanostructured materials have also focused on enhancing the durability and longevity of proton-conducting systems. The harsh operating conditions of fuel cells, which often involve high temperatures and acidic environments, pose significant challenges to the stability of these materials. However, by engineering nanostructures with robust chemical bonds and protective coatings, researchers have been able to extend the lifespan of proton-conducting materials, thereby reducing the need for frequent replacements and maintenance. This not only lowers the overall cost of energy systems but also contributes to their environmental sustainability.
Furthermore, the versatility of nanostructured proton-conducting materials has enabled their application in a wide range of energy technologies beyond fuel cells. For instance, these materials are being explored for use in electrochemical sensors, supercapacitors, and even in the development of next-generation batteries. The ability to tailor the properties of nanostructured materials to meet the specific requirements of different applications underscores their potential as a key enabler of future green energy solutions.
In conclusion, the advances in nanostructured proton-conducting materials represent a significant leap forward in the quest for efficient and sustainable energy technologies. By enhancing proton conductivity, durability, and versatility, these materials are paving the way for innovative solutions that could transform the energy landscape. As research in this field continues to progress, it is expected that nanostructured proton-conducting materials will play an increasingly vital role in the transition towards a more sustainable and environmentally friendly energy future.
Challenges and Opportunities in the Development of Proton-Conducting Electrolytes
The development of proton-conducting electrolytes represents a significant frontier in the quest for innovative green energy solutions. As the world increasingly shifts towards sustainable energy sources, the demand for efficient and environmentally friendly technologies has never been more pressing. Proton-conducting materials, particularly those used in fuel cells, offer a promising avenue for clean energy production. However, the journey towards their widespread implementation is fraught with both challenges and opportunities that must be navigated with precision and foresight.
One of the primary challenges in the development of proton-conducting electrolytes is achieving high proton conductivity at low temperatures. Traditional materials often require elevated temperatures to facilitate efficient proton transport, which can lead to increased energy consumption and reduced overall efficiency. This necessitates the exploration of novel materials that can operate effectively at ambient conditions. Researchers are actively investigating various classes of materials, such as perovskites, metal-organic frameworks, and polymer-based electrolytes, each offering unique properties that could potentially overcome this hurdle. The synthesis and characterization of these materials require sophisticated techniques and a deep understanding of their structural and chemical properties.
In addition to conductivity, the chemical and thermal stability of proton-conducting materials is a critical factor that influences their practical application. Many potential electrolytes suffer from degradation under operational conditions, which can lead to a loss of performance and a shortened lifespan of the fuel cell. This challenge presents an opportunity for material scientists to innovate by designing materials that not only exhibit high conductivity but also maintain stability over prolonged periods. Advanced computational modeling and experimental methods are being employed to predict and enhance the durability of these materials, thereby paving the way for more reliable and long-lasting energy solutions.
Moreover, the scalability and cost-effectiveness of producing proton-conducting materials are significant considerations that impact their commercial viability. While laboratory-scale experiments may yield promising results, translating these findings into large-scale production often presents unforeseen difficulties. The synthesis processes must be optimized to ensure that they are economically feasible and environmentally sustainable. This requires a multidisciplinary approach, combining insights from chemistry, engineering, and economics to develop scalable production methods that do not compromise the material’s performance.
Despite these challenges, the opportunities presented by proton-conducting materials are substantial. Their potential to revolutionize the energy sector is underscored by their application in hydrogen fuel cells, which offer a clean alternative to fossil fuels. By converting hydrogen into electricity with water as the only byproduct, these cells represent a pivotal step towards reducing carbon emissions and combating climate change. Furthermore, advancements in proton-conducting materials could extend beyond fuel cells, impacting other areas such as sensors, electrochemical devices, and even medical applications.
In conclusion, while the development of proton-conducting electrolytes is accompanied by significant challenges, the opportunities they present for advancing green energy solutions are equally compelling. Through continued research and innovation, it is possible to overcome the obstacles of conductivity, stability, and scalability, ultimately leading to the widespread adoption of these materials. As the global community strives towards a more sustainable future, proton-conducting materials stand at the forefront of this transformation, offering a glimpse into a world powered by clean and efficient energy technologies.
Future Trends in Proton-Conducting Materials for Renewable Energy Solutions
Proton-conducting materials have emerged as a pivotal component in the quest for innovative green energy solutions, offering promising avenues for the development of efficient and sustainable energy systems. As the global demand for clean energy continues to rise, the exploration of advanced materials that can facilitate proton conduction is becoming increasingly critical. These materials are integral to the operation of fuel cells, which are devices that convert chemical energy into electrical energy through electrochemical reactions. Fuel cells, particularly proton exchange membrane fuel cells (PEMFCs), are gaining traction due to their high efficiency and low environmental impact, making the study of proton-conducting materials a focal point in renewable energy research.
The future trends in proton-conducting materials are largely driven by the need to enhance the performance, durability, and cost-effectiveness of fuel cells. One of the primary challenges in this field is the development of materials that can operate efficiently at a wide range of temperatures and humidity levels. Traditional proton-conducting materials, such as Nafion, have limitations in terms of their operational temperature range and cost. Consequently, researchers are exploring alternative materials, including polymers, ceramics, and composite materials, that can overcome these limitations. For instance, the development of high-temperature proton conductors is a promising area of research, as these materials can potentially improve the efficiency and longevity of fuel cells by reducing the need for complex thermal management systems.
In addition to temperature resilience, the future of proton-conducting materials is also focused on enhancing their mechanical and chemical stability. The harsh operating conditions within fuel cells, such as acidic environments and high mechanical stress, necessitate materials that can maintain their structural integrity over prolonged periods. To address this, researchers are investigating the incorporation of nanomaterials and hybrid structures that can provide the necessary robustness while maintaining high proton conductivity. These innovations are expected to lead to the next generation of fuel cells that are not only more durable but also more adaptable to various applications, from portable electronics to large-scale power generation.
Moreover, the sustainability of proton-conducting materials is a growing concern, prompting the exploration of eco-friendly and cost-effective alternatives. The synthesis of bio-based polymers and the utilization of abundant natural resources are gaining attention as viable strategies to reduce the environmental footprint of these materials. By leveraging green chemistry principles, researchers aim to develop proton conductors that are not only efficient but also align with the broader goals of sustainability and resource conservation.
As the field of proton-conducting materials continues to evolve, interdisciplinary collaboration is becoming increasingly important. The integration of insights from materials science, chemistry, and engineering is essential to address the complex challenges associated with the development of advanced proton conductors. Furthermore, partnerships between academia, industry, and government agencies are crucial to accelerate the translation of research findings into practical applications.
In conclusion, the future trends in proton-conducting materials for renewable energy solutions are characterized by a focus on enhancing performance, durability, and sustainability. Through innovative research and collaborative efforts, these materials hold the potential to revolutionize the landscape of green energy technologies, paving the way for a cleaner and more sustainable future. As advancements continue to unfold, the role of proton-conducting materials in shaping the future of energy cannot be overstated, offering a beacon of hope in the global pursuit of sustainable energy solutions.
Q&A
1. **What are proton-conducting materials?**
Proton-conducting materials are substances that facilitate the movement of protons (H⁺ ions) through their structure, making them essential components in devices like fuel cells and electrolyzers.
2. **Why are proton-conducting materials important for green energy?**
They are crucial for green energy because they enable efficient energy conversion and storage in fuel cells, which can generate electricity with water as the only byproduct, thus reducing carbon emissions.
3. **What are some common types of proton-conducting materials?**
Common types include perfluorosulfonic acid polymers (e.g., Nafion), ceramic oxides (e.g., barium zirconate), and organic-inorganic hybrid materials.
4. **How do proton-conducting materials work in fuel cells?**
In fuel cells, these materials act as electrolytes, allowing protons generated from hydrogen at the anode to move to the cathode, where they combine with oxygen to produce water and electricity.
5. **What are the challenges in developing proton-conducting materials?**
Challenges include improving conductivity at lower temperatures, enhancing chemical and mechanical stability, and reducing costs for large-scale applications.
6. **What innovations are being explored in proton-conducting materials?**
Innovations include developing new polymer blends, designing nanocomposites, and exploring alternative materials like metal-organic frameworks (MOFs) to improve performance and durability.Proton-conducting materials are at the forefront of advancing green energy technologies, offering significant potential for sustainable and efficient energy solutions. These materials, which facilitate the movement of protons, are crucial in the development of fuel cells, electrolyzers, and other energy conversion devices. Their ability to operate at lower temperatures and with high efficiency makes them ideal for reducing the carbon footprint associated with traditional energy systems. Innovations in the synthesis and design of proton-conducting materials are enhancing their conductivity, stability, and durability, thereby improving the performance and cost-effectiveness of green energy technologies. As research progresses, these materials are expected to play a pivotal role in the transition to a cleaner energy future, supporting global efforts to mitigate climate change and reduce reliance on fossil fuels.
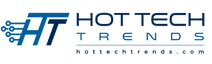