Unraveling the intricate tapestry of protein evolution offers profound insights into the origins of life on Earth. Proteins, the workhorses of cellular machinery, are fundamental to understanding how life has diversified and adapted over billions of years. By tracing the evolutionary pathways of proteins, scientists can reconstruct the molecular events that led to the emergence of complex life forms from simple ancestral origins. This exploration not only sheds light on the biochemical innovations that have driven evolutionary success but also provides a window into the primordial conditions that fostered the genesis of life. Through advanced techniques in molecular biology, bioinformatics, and evolutionary biology, researchers are piecing together the evolutionary history encoded in protein structures and sequences, offering a glimpse into the ancient biological processes that continue to shape life today.
Understanding Protein Evolution: A Journey Through Time
Understanding protein evolution is a fascinating journey that takes us back to the very origins of life on Earth. Proteins, the workhorses of the cell, are complex molecules that perform a myriad of functions essential for life. They are composed of long chains of amino acids, and their specific sequences determine their structure and function. The study of protein evolution not only provides insights into how life has adapted and diversified over billions of years but also offers clues about the earliest forms of life.
To comprehend protein evolution, it is crucial to consider the role of genetic mutations. Mutations are changes in the DNA sequence that can lead to variations in protein structure and function. Over time, these mutations accumulate, and through the process of natural selection, advantageous mutations are preserved, while deleterious ones are eliminated. This gradual accumulation of beneficial mutations leads to the evolution of new proteins with novel functions, allowing organisms to adapt to changing environments.
The origins of proteins can be traced back to the prebiotic world, where simple organic molecules formed under the conditions of early Earth. It is hypothesized that these molecules eventually gave rise to the first amino acids, which then linked together to form primitive proteins. These early proteins were likely simple and unstable, but they provided a foundation for the development of more complex proteins. As life evolved, so did the mechanisms for protein synthesis, leading to the sophisticated machinery we observe in modern cells.
One of the key methods for studying protein evolution is the comparison of protein sequences across different species. By analyzing these sequences, scientists can infer evolutionary relationships and identify conserved regions that have remained unchanged over millions of years. These conserved regions often correspond to critical functional domains, highlighting their importance in maintaining protein function. Additionally, the study of protein structures can reveal how evolutionary pressures have shaped the three-dimensional conformation of proteins, further elucidating their functional roles.
The evolution of proteins is also closely linked to the emergence of new biological functions. Gene duplication events, where a gene is copied within the genome, provide raw material for evolutionary innovation. The duplicated gene can acquire mutations that lead to a new function, while the original gene retains its original role. This process, known as neofunctionalization, has been a driving force in the diversification of life, allowing organisms to exploit new ecological niches.
Moreover, horizontal gene transfer, the movement of genetic material between unrelated species, has played a significant role in protein evolution. This process allows for the rapid acquisition of new genes and functions, facilitating adaptation to new environments. It is particularly prevalent in microorganisms, where it contributes to the spread of antibiotic resistance and other adaptive traits.
In conclusion, the study of protein evolution provides a window into the history of life on Earth. By unraveling the complex processes that have shaped proteins over billions of years, scientists can gain a deeper understanding of how life has evolved and adapted. This knowledge not only enhances our understanding of biology but also has practical applications in fields such as medicine and biotechnology, where insights into protein function and evolution can inform the development of new therapies and technologies. As research in this area continues to advance, it promises to shed further light on the intricate tapestry of life and its origins.
The Role of Proteins in Early Life Forms
Proteins, as fundamental components of all living organisms, play a crucial role in the myriad processes that sustain life. Understanding their evolution offers profound insights into the origins of life itself. In the earliest life forms, proteins were not only structural elements but also catalysts for biochemical reactions, enabling the complex interplay of molecules necessary for life. As we delve into the role of proteins in early life forms, it becomes evident that their evolution is intricately linked to the emergence and diversification of life on Earth.
Initially, proteins likely emerged from simpler molecules in the primordial soup, a theoretical mixture of organic compounds present on the early Earth. These early proteins, or proto-proteins, were likely composed of a limited set of amino acids, the building blocks of proteins. Over time, through processes such as natural selection and genetic mutation, these proto-proteins evolved into more complex structures, capable of performing a wider range of functions. This evolutionary process was crucial for the development of more sophisticated life forms, as proteins began to specialize in various roles, from catalyzing metabolic reactions to forming cellular structures.
The versatility of proteins is largely due to their diverse structures, which are determined by the sequence of amino acids. This sequence dictates how a protein folds into its three-dimensional shape, which in turn influences its function. In early life forms, the ability of proteins to adopt different shapes and functions was a key factor in their survival and adaptation to changing environments. For instance, enzymes, a class of proteins that act as catalysts, were essential for accelerating chemical reactions that would otherwise occur too slowly to sustain life. The evolution of enzymes allowed early organisms to efficiently harness energy and resources from their surroundings, facilitating their growth and reproduction.
Moreover, proteins played a pivotal role in the development of cellular membranes, which are essential for maintaining the integrity and functionality of cells. Membrane proteins, embedded within the lipid bilayer of cell membranes, are responsible for a variety of functions, including transport of molecules, signal transduction, and cell communication. In early life forms, the evolution of membrane proteins was crucial for the establishment of cellular compartments, which allowed for the segregation of different biochemical processes and the creation of distinct cellular environments.
As life continued to evolve, proteins became increasingly complex, with new functions emerging through the duplication and divergence of existing proteins. This process, known as gene duplication, allowed organisms to experiment with new protein functions without losing the original function. Over time, this led to the vast diversity of proteins observed in modern organisms, each tailored to specific roles within the cell.
In conclusion, the role of proteins in early life forms was instrumental in shaping the trajectory of life’s evolution. From their humble beginnings as simple chains of amino acids, proteins have evolved into highly specialized molecules that underpin the complexity of life. By tracing the evolution of proteins, scientists can gain valuable insights into the origins of life and the mechanisms that have driven its diversification over billions of years. As research in this field continues to advance, it holds the promise of unraveling the mysteries of life’s earliest beginnings and the fundamental processes that sustain it.
Molecular Clocks: Dating the Origins of Proteins
The study of protein evolution offers a fascinating glimpse into the origins of life, providing insights into how complex biological systems have developed over billions of years. Central to this exploration is the concept of molecular clocks, a method that allows scientists to estimate the timing of evolutionary events by analyzing genetic mutations. These molecular clocks are based on the premise that genetic mutations accumulate at relatively constant rates over time, thus serving as a chronological tool to trace the lineage of proteins back to their ancestral forms.
To understand the origins of proteins, researchers first identify homologous proteins across different species. These proteins, which share a common ancestry, can be compared to determine the number of genetic differences that have arisen since their divergence from a common ancestor. By calibrating these differences with known fossil records or other historical data, scientists can estimate the rate of mutation and, consequently, the time at which these proteins first appeared. This approach has been instrumental in constructing phylogenetic trees, which map the evolutionary relationships between species and their proteins.
However, the application of molecular clocks is not without its challenges. One significant issue is the assumption of a constant mutation rate, which may not hold true across all lineages or environmental conditions. Factors such as natural selection, genetic drift, and varying environmental pressures can influence mutation rates, leading to discrepancies in molecular clock estimates. To address these challenges, researchers often employ sophisticated statistical models that account for rate variation, thereby refining the accuracy of their evolutionary timelines.
Despite these complexities, molecular clocks have yielded remarkable insights into the history of life on Earth. For instance, they have helped pinpoint the emergence of key proteins involved in fundamental biological processes, such as photosynthesis and cellular respiration. By dating these proteins, scientists can infer the timing of major evolutionary transitions, such as the rise of oxygenic photosynthesis, which dramatically altered Earth’s atmosphere and paved the way for the evolution of complex life forms.
Moreover, molecular clocks have shed light on the origins of specific protein families, such as enzymes and structural proteins, which play crucial roles in cellular function. By tracing the evolutionary history of these proteins, researchers can better understand how they have adapted to meet the diverse needs of different organisms. This knowledge not only enriches our understanding of evolutionary biology but also has practical applications in fields such as medicine and biotechnology, where insights into protein function and evolution can inform drug development and the design of novel biomolecules.
In addition to their role in dating the origins of proteins, molecular clocks have also contributed to our understanding of the broader evolutionary landscape. By integrating molecular data with paleontological and geological evidence, scientists can construct a more comprehensive picture of life’s history, revealing patterns of diversification and extinction that have shaped the biosphere over time. This interdisciplinary approach underscores the importance of molecular clocks as a tool for unraveling the complex tapestry of life on Earth.
In conclusion, the study of protein evolution through molecular clocks offers a window into the deep past, allowing us to trace the origins of life and the intricate web of relationships that connect all living organisms. While challenges remain in refining these methods, the insights gained from this research continue to illuminate the pathways of evolution, enhancing our understanding of the natural world and our place within it.
Evolutionary Mechanisms: How Proteins Adapt and Change
Proteins, the workhorses of the cell, are fundamental to the myriad processes that sustain life. Understanding how proteins evolve offers profound insights into the mechanisms of evolution and the origins of life itself. The journey of protein evolution is a complex interplay of genetic mutations, natural selection, and environmental pressures, all of which contribute to the diversity and functionality of proteins observed today. As we delve into the evolutionary mechanisms that drive protein adaptation and change, it becomes evident that these processes are both intricate and fascinating.
To begin with, the primary mechanism by which proteins evolve is through genetic mutations. These mutations occur at the level of DNA, where changes in nucleotide sequences can lead to alterations in the amino acid composition of proteins. While many mutations are neutral or deleterious, some confer advantageous traits that enhance an organism’s survival and reproductive success. Over time, beneficial mutations are preserved through natural selection, leading to the gradual evolution of protein structures and functions. This process is not random but rather a directed response to the challenges and opportunities presented by the environment.
Moreover, the concept of gene duplication plays a pivotal role in protein evolution. When a gene is duplicated, it provides an additional copy that is free to accumulate mutations without compromising the original gene’s function. This redundancy allows for the exploration of new functions and the emergence of novel proteins. Gene duplication has been instrumental in the evolution of complex organisms, as it facilitates the diversification of protein families and the development of specialized functions. For instance, the evolution of hemoglobin from ancestral globin proteins illustrates how gene duplication and subsequent divergence can lead to proteins with distinct and essential roles in physiology.
In addition to genetic mutations and gene duplication, horizontal gene transfer is another mechanism that contributes to protein evolution. This process involves the transfer of genetic material between unrelated species, allowing for the rapid acquisition of new traits. Horizontal gene transfer is particularly prevalent in prokaryotes, where it can lead to the spread of antibiotic resistance and other adaptive traits. By incorporating foreign genes, organisms can quickly adapt to new environments and challenges, highlighting the dynamic nature of protein evolution.
Furthermore, the role of environmental pressures cannot be overstated in shaping protein evolution. Proteins must adapt to a wide range of conditions, from extreme temperatures to varying pH levels, to maintain their functionality. These adaptations often involve changes in protein stability, folding, and interactions with other molecules. For example, extremophiles, organisms that thrive in harsh environments, have evolved proteins with unique structural features that confer stability under extreme conditions. Such adaptations underscore the importance of environmental factors in driving the evolutionary trajectory of proteins.
In conclusion, the evolution of proteins is a multifaceted process that is central to the diversity of life on Earth. Through genetic mutations, gene duplication, horizontal gene transfer, and environmental pressures, proteins have adapted and changed over billions of years. These evolutionary mechanisms not only illuminate the origins of life but also provide a framework for understanding how organisms continue to evolve in response to an ever-changing world. As research in this field advances, it promises to unravel further the complexities of protein evolution and its implications for biology and medicine.
Ancestral Protein Reconstruction: Insights into Ancient Life
Ancestral protein reconstruction has emerged as a fascinating field of study, offering profound insights into the molecular underpinnings of ancient life. By delving into the evolutionary history of proteins, scientists are able to piece together the complex puzzle of life’s origins. This approach not only enhances our understanding of how life evolved on Earth but also provides a window into the biochemical capabilities of early organisms. As researchers continue to refine their techniques, the reconstruction of ancestral proteins is shedding light on the evolutionary pressures and environmental conditions that shaped the trajectory of life.
The process of ancestral protein reconstruction involves inferring the sequences of ancient proteins by analyzing the genetic information of their modern descendants. This is achieved through sophisticated computational algorithms that trace the evolutionary lineage of proteins, allowing scientists to predict the most likely sequences of their common ancestors. Once these sequences are hypothesized, they can be synthesized in the laboratory, enabling researchers to study their properties and functions. This experimental approach provides a tangible link to the past, offering a glimpse into the molecular machinery that powered early life forms.
One of the key insights gained from ancestral protein reconstruction is the adaptability and resilience of early life. By examining the biochemical properties of reconstructed proteins, scientists have discovered that ancient proteins often exhibit remarkable stability and functionality under a wide range of conditions. This suggests that early life forms were equipped to survive in the harsh and fluctuating environments of primordial Earth. Moreover, the study of these proteins has revealed how evolutionary innovations, such as the development of new enzymatic activities, allowed organisms to exploit new ecological niches and adapt to changing environments.
Furthermore, ancestral protein reconstruction has provided valuable information about the origins of complex biological systems. For instance, by reconstructing the proteins involved in photosynthesis, researchers have gained insights into how this critical process evolved. The ability to harness sunlight for energy was a pivotal development in the history of life, and understanding its origins helps elucidate the evolutionary pressures that drove the diversification of life on Earth. Similarly, the study of ancient proteins involved in cellular respiration has shed light on the evolution of metabolic pathways, revealing how early organisms optimized energy production to sustain life.
In addition to offering insights into the past, ancestral protein reconstruction has practical applications in modern science. By understanding the properties of ancient proteins, researchers can design novel proteins with enhanced stability and functionality for use in biotechnology and medicine. This approach, known as protein engineering, holds promise for the development of new therapeutics and industrial enzymes, highlighting the relevance of evolutionary studies to contemporary challenges.
As the field of ancestral protein reconstruction continues to advance, it is poised to answer fundamental questions about the origins and evolution of life. By bridging the gap between the past and present, this research not only enriches our understanding of life’s history but also informs our approach to addressing future scientific and technological challenges. The study of ancient proteins serves as a testament to the power of evolution, illustrating how the intricate dance of molecular adaptation has shaped the diversity of life we see today. Through the lens of ancestral protein reconstruction, we gain a deeper appreciation for the complexity and resilience of life, tracing its roots back to the dawn of existence.
The Impact of Protein Evolution on Modern Biology
The study of protein evolution has profoundly impacted modern biology, offering insights into the fundamental processes that have shaped life on Earth. Proteins, as the workhorses of the cell, are involved in virtually every biological function, from catalyzing metabolic reactions to providing structural support. Understanding how proteins have evolved over billions of years not only illuminates the history of life but also enhances our ability to manipulate biological systems for medical and technological advancements.
Tracing the evolutionary history of proteins involves examining the sequences of amino acids that compose them. These sequences are encoded by genes, and changes in these sequences over time, driven by mutations, natural selection, and genetic drift, have led to the vast diversity of proteins observed today. By comparing protein sequences across different species, scientists can infer evolutionary relationships and identify conserved regions that are critical for protein function. This comparative approach has been instrumental in constructing phylogenetic trees, which depict the evolutionary pathways of organisms and their proteins.
The impact of protein evolution extends beyond understanding life’s history; it also provides a framework for studying the mechanisms of evolution itself. For instance, the concept of molecular evolution, which focuses on changes at the molecular level, has been pivotal in elucidating how proteins adapt to new functions or environments. This adaptability is often achieved through a process known as gene duplication, where a gene is copied, and the duplicate can evolve new functions while the original maintains its role. Such events have been crucial in the evolution of complex organisms, allowing for the development of novel traits and increased biological complexity.
Moreover, protein evolution has significant implications for modern medicine. Many diseases, including cancer and genetic disorders, are linked to mutations in proteins that alter their function. By understanding the evolutionary history of these proteins, researchers can identify critical regions that are prone to harmful mutations and develop targeted therapies. For example, the study of viral protein evolution has been essential in tracking the emergence of new viral strains and developing vaccines. The rapid evolution of viral proteins, such as those in the influenza virus or SARS-CoV-2, poses challenges for vaccine design, but evolutionary insights can guide the development of more effective and broad-spectrum vaccines.
In addition to its medical applications, protein evolution is a cornerstone of biotechnology. The ability to engineer proteins with desired properties relies heavily on understanding how proteins have evolved to perform specific functions. Techniques such as directed evolution mimic natural selection in the laboratory, allowing scientists to evolve proteins with enhanced stability, activity, or specificity. This approach has led to the creation of enzymes used in industrial processes, environmentally friendly catalysts, and novel therapeutics.
Furthermore, the study of protein evolution is increasingly relevant in the context of synthetic biology, where the goal is to design and construct new biological systems. By leveraging evolutionary principles, researchers can create proteins with functions not found in nature, opening up possibilities for innovative solutions to global challenges, such as sustainable energy production and environmental remediation.
In conclusion, the impact of protein evolution on modern biology is profound and multifaceted. By unraveling the evolutionary history of proteins, scientists gain insights into the origins and diversification of life, the mechanisms of evolution, and the potential for future innovations in medicine and biotechnology. As research in this field continues to advance, it promises to further illuminate the intricate tapestry of life and enhance our ability to harness biological systems for the betterment of society.
Q&A
1. **What is protein evolution?**
Protein evolution refers to the process by which protein structures and functions change over time through genetic mutations, natural selection, and other evolutionary mechanisms.
2. **Why is understanding protein evolution important?**
Understanding protein evolution is crucial for insights into how life originated and diversified, how proteins acquire new functions, and how they can be engineered for medical and industrial applications.
3. **How do scientists study protein evolution?**
Scientists study protein evolution through comparative genomics, structural biology, and bioinformatics, analyzing protein sequences and structures across different species to infer evolutionary relationships.
4. **What role do mutations play in protein evolution?**
Mutations introduce changes in the amino acid sequence of proteins, which can alter their structure and function, providing raw material for natural selection to act upon.
5. **How can protein evolution help trace back to life’s origins?**
By reconstructing ancestral proteins and studying their properties, scientists can infer the characteristics of early life forms and the environmental conditions of early Earth.
6. **What are some challenges in studying protein evolution?**
Challenges include the complexity of protein structures, the vast diversity of proteins, incomplete fossil records, and the difficulty of experimentally validating evolutionary hypotheses.Unraveling protein evolution provides critical insights into the origins of life by tracing the structural and functional changes that proteins have undergone over billions of years. By examining ancient protein sequences and reconstructing ancestral proteins, scientists can infer the environmental conditions and biological processes that shaped early life on Earth. This research not only enhances our understanding of how complex biological systems evolved from simple molecules but also sheds light on the fundamental principles of molecular evolution. Ultimately, studying protein evolution helps bridge the gap between chemistry and biology, offering a clearer picture of how life emerged and adapted to diverse environments over time.
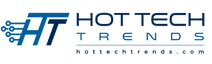