Pioneering breakthroughs in drug and medicine development have revolutionized the landscape of healthcare, offering unprecedented opportunities to combat diseases and improve patient outcomes. These advancements are the result of relentless research, cutting-edge technology, and innovative methodologies that have transformed the way we understand and treat various medical conditions. From the discovery of novel therapeutic targets to the development of personalized medicine, the field has witnessed significant progress that has not only enhanced the efficacy and safety of treatments but also expanded the horizons of what is possible in medical science. As we continue to explore the complexities of human biology and disease, these breakthroughs hold the promise of ushering in a new era of precision medicine, where treatments are tailored to the individual needs of patients, ultimately leading to more effective and sustainable healthcare solutions.
Revolutionary Gene Editing Techniques in Medicine
In recent years, the field of medicine has witnessed remarkable advancements, particularly in the realm of gene editing techniques. These pioneering breakthroughs have opened new avenues for treating a myriad of genetic disorders, offering hope to millions of patients worldwide. At the forefront of these innovations is CRISPR-Cas9, a revolutionary tool that has transformed the landscape of genetic research and therapeutic applications. By allowing precise modifications to DNA sequences, CRISPR-Cas9 has enabled scientists to target and correct genetic mutations with unprecedented accuracy. This has significant implications for the treatment of hereditary diseases, such as cystic fibrosis, sickle cell anemia, and muscular dystrophy, where faulty genes can now be directly addressed.
Moreover, the versatility of CRISPR-Cas9 extends beyond correcting genetic defects. It has also been instrumental in advancing cancer research, where it is used to identify and disable genes that contribute to tumor growth and resistance to treatment. By understanding the genetic underpinnings of cancer, researchers can develop more effective and personalized therapies, potentially transforming the prognosis for patients with various forms of the disease. Furthermore, CRISPR-Cas9 has facilitated the development of novel immunotherapies, such as CAR-T cell therapy, which involves engineering a patient’s immune cells to better recognize and attack cancer cells.
In addition to CRISPR-Cas9, other gene editing technologies, such as base editing and prime editing, have emerged, each offering unique advantages. Base editing allows for the conversion of one DNA base pair into another without causing double-strand breaks, reducing the risk of unintended mutations. This technique is particularly useful for correcting point mutations, which are responsible for a significant proportion of genetic disorders. Prime editing, on the other hand, offers even greater precision by enabling the insertion, deletion, or replacement of DNA sequences without the need for donor DNA templates. These advancements highlight the rapid evolution of gene editing tools and their potential to address a broader range of genetic conditions.
Despite these promising developments, the application of gene editing in medicine is not without challenges. Ethical considerations, such as the potential for unintended genetic modifications and the long-term effects of gene editing, must be carefully weighed. Additionally, the delivery of gene editing tools to specific cells and tissues remains a significant hurdle. Researchers are actively exploring various delivery methods, including viral vectors and nanoparticles, to enhance the efficiency and safety of gene editing therapies.
As the field continues to evolve, collaboration between scientists, clinicians, and regulatory bodies will be crucial in ensuring the responsible development and implementation of gene editing technologies. Rigorous clinical trials and comprehensive risk assessments will be essential to establish the safety and efficacy of these therapies before they can be widely adopted in clinical practice.
In conclusion, revolutionary gene editing techniques have ushered in a new era of medicine, offering unprecedented opportunities to treat and potentially cure genetic disorders. While challenges remain, the continued refinement of these technologies and their integration into clinical practice hold the promise of transforming healthcare and improving the lives of countless individuals. As we stand on the cusp of this genetic revolution, the potential for groundbreaking discoveries and therapeutic innovations is immense, heralding a future where genetic diseases may no longer be an insurmountable obstacle.
The Rise of Personalized Medicine and Its Impact
The field of drug and medicine development has witnessed remarkable advancements over the past few decades, with personalized medicine emerging as a transformative approach that is reshaping the landscape of healthcare. Personalized medicine, also known as precision medicine, tailors medical treatment to the individual characteristics of each patient, taking into account genetic, environmental, and lifestyle factors. This innovative approach is not only enhancing the efficacy of treatments but also minimizing adverse effects, thereby significantly improving patient outcomes.
One of the key drivers behind the rise of personalized medicine is the rapid advancement in genomic technologies. The completion of the Human Genome Project in 2003 marked a pivotal moment, providing researchers with a comprehensive map of human DNA. This breakthrough has enabled scientists to identify genetic variations that contribute to disease susceptibility and drug response. Consequently, healthcare providers can now utilize genetic testing to predict how patients will respond to specific medications, allowing for more informed and effective treatment decisions.
Moreover, the integration of big data analytics and artificial intelligence (AI) into healthcare has further accelerated the development of personalized medicine. By analyzing vast amounts of data from diverse sources, such as electronic health records, clinical trials, and wearable devices, AI algorithms can identify patterns and correlations that were previously undetectable. This capability allows for the development of predictive models that can forecast disease progression and treatment outcomes, thereby facilitating the design of personalized therapeutic strategies.
In addition to genetic and data-driven approaches, personalized medicine also encompasses the use of biomarkers—biological molecules that indicate normal or abnormal processes in the body. Biomarkers can be used to diagnose diseases, predict disease progression, and monitor treatment responses. For instance, in oncology, the identification of specific biomarkers has led to the development of targeted therapies that attack cancer cells with precision, sparing healthy cells and reducing side effects. This has been particularly beneficial in treating cancers such as breast, lung, and melanoma, where traditional chemotherapy often falls short.
The impact of personalized medicine extends beyond individual patient care, influencing the broader healthcare system as well. By optimizing treatment regimens, personalized medicine can reduce the incidence of trial-and-error prescribing, which often leads to unnecessary healthcare costs and patient suffering. Furthermore, it holds the potential to streamline drug development processes by identifying patient subgroups that are more likely to benefit from new therapies, thereby increasing the success rates of clinical trials.
Despite its promising potential, the implementation of personalized medicine faces several challenges. Ethical considerations, such as patient privacy and data security, must be addressed to ensure that sensitive genetic information is protected. Additionally, the high costs associated with genetic testing and advanced therapies may limit accessibility for some patients, raising concerns about healthcare equity. To overcome these barriers, collaboration among stakeholders, including researchers, healthcare providers, policymakers, and industry leaders, is essential.
In conclusion, the rise of personalized medicine represents a paradigm shift in drug and medicine development, offering the promise of more precise, effective, and patient-centered healthcare. As technological advancements continue to unfold, the integration of personalized medicine into routine clinical practice is likely to expand, ultimately transforming the way diseases are diagnosed, treated, and prevented. By embracing this innovative approach, the healthcare community can pave the way for a future where medical interventions are not only more effective but also more attuned to the unique needs of each individual.
Breakthroughs in Immunotherapy for Cancer Treatment
Immunotherapy has emerged as a groundbreaking approach in the fight against cancer, offering new hope to patients and transforming the landscape of oncology. This innovative treatment harnesses the body’s immune system to identify and combat cancer cells, marking a significant departure from traditional methods such as chemotherapy and radiation. As research in this field advances, several pioneering breakthroughs have been made, each contributing to the efficacy and applicability of immunotherapy in cancer treatment.
One of the most notable advancements in immunotherapy is the development of immune checkpoint inhibitors. These drugs work by blocking proteins that prevent the immune system from attacking cancer cells. By inhibiting these checkpoints, the immune system can recognize and destroy cancerous cells more effectively. The introduction of checkpoint inhibitors such as pembrolizumab and nivolumab has revolutionized the treatment of various cancers, including melanoma, lung cancer, and renal cell carcinoma. Clinical trials have demonstrated significant improvements in patient survival rates, underscoring the potential of these drugs to change the course of cancer treatment.
In addition to checkpoint inhibitors, another promising area of immunotherapy is the use of CAR T-cell therapy. This personalized treatment involves modifying a patient’s T-cells to better recognize and attack cancer cells. Once reintroduced into the patient’s body, these engineered T-cells can target and eliminate cancer with remarkable precision. CAR T-cell therapy has shown exceptional success in treating certain types of blood cancers, such as acute lymphoblastic leukemia and non-Hodgkin lymphoma. The success of this approach has spurred further research into expanding its application to solid tumors, which presents a more complex challenge due to the tumor microenvironment.
Moreover, the exploration of cancer vaccines represents another frontier in immunotherapy. Unlike traditional vaccines that prevent disease, cancer vaccines aim to treat existing cancer by stimulating the immune system to attack cancer cells. These vaccines can be tailored to target specific antigens present on cancer cells, thereby enhancing the immune response. While still in the experimental stages, cancer vaccines have shown promise in clinical trials, particularly in combination with other immunotherapies, suggesting a synergistic effect that could enhance treatment outcomes.
Furthermore, the integration of advanced technologies such as artificial intelligence and genomics is accelerating the pace of discovery in immunotherapy. By analyzing vast amounts of genetic data, researchers can identify novel targets for immunotherapy and predict patient responses to treatment. This precision medicine approach allows for more personalized and effective treatment strategies, minimizing adverse effects and maximizing therapeutic benefits.
Despite these remarkable advancements, challenges remain in the widespread adoption of immunotherapy. Issues such as treatment resistance, high costs, and the management of immune-related side effects continue to pose significant hurdles. However, ongoing research and collaboration among scientists, clinicians, and pharmaceutical companies are paving the way for overcoming these obstacles.
In conclusion, the breakthroughs in immunotherapy for cancer treatment represent a paradigm shift in oncology, offering new avenues for combating a disease that has long eluded a cure. As research continues to unravel the complexities of the immune system and its interaction with cancer, the potential for immunotherapy to provide durable and effective treatment options becomes increasingly attainable. The future of cancer treatment is undoubtedly being reshaped by these pioneering efforts, bringing hope to millions of patients worldwide.
The Role of Artificial Intelligence in Drug Discovery
In recent years, the field of drug discovery has witnessed a transformative shift, largely driven by the integration of artificial intelligence (AI) into its processes. This technological advancement has not only accelerated the pace of drug development but also enhanced the precision and efficacy of new therapeutic solutions. As the pharmaceutical industry grapples with the challenges of high costs and lengthy timelines associated with traditional drug discovery methods, AI emerges as a beacon of innovation, offering promising solutions to these enduring issues.
To begin with, AI’s ability to process vast amounts of data with remarkable speed and accuracy is revolutionizing the initial stages of drug discovery. Traditionally, identifying potential drug candidates involved labor-intensive and time-consuming laboratory experiments. However, AI algorithms can now analyze extensive datasets, including genomic information, chemical properties, and biological interactions, to predict which compounds are most likely to succeed as effective drugs. This data-driven approach not only reduces the time required to identify viable candidates but also minimizes the risk of costly failures in later stages of development.
Moreover, AI’s role extends beyond mere data analysis. Machine learning models, a subset of AI, are being employed to simulate complex biological processes and predict how different compounds will interact with specific targets in the human body. This capability is particularly valuable in the design of personalized medicine, where treatments are tailored to the unique genetic makeup of individual patients. By leveraging AI, researchers can develop more targeted therapies, thereby increasing the likelihood of successful outcomes and reducing adverse side effects.
In addition to enhancing the discovery phase, AI is also making significant contributions to the optimization of clinical trials. Traditionally, clinical trials are fraught with challenges, including patient recruitment, trial design, and data management. AI can streamline these processes by identifying suitable candidates for trials through the analysis of electronic health records and other relevant data sources. Furthermore, AI-driven predictive analytics can optimize trial design by simulating various scenarios and identifying the most effective strategies for testing new drugs. This not only expedites the trial process but also improves the reliability of the results obtained.
Furthermore, AI’s impact on drug discovery is not limited to the development of new drugs. It is also playing a crucial role in drug repurposing, where existing medications are evaluated for new therapeutic uses. By analyzing existing data on approved drugs, AI can identify potential new applications, thereby extending the lifecycle of these drugs and offering new treatment options for patients. This approach is particularly valuable in addressing urgent health crises, such as the COVID-19 pandemic, where time is of the essence in finding effective treatments.
Despite the numerous advantages AI brings to drug discovery, it is important to acknowledge the challenges and limitations that accompany its integration. Issues such as data privacy, algorithmic bias, and the need for interdisciplinary collaboration must be addressed to fully harness the potential of AI in this field. Nevertheless, the ongoing advancements in AI technology and its increasing adoption in pharmaceutical research signal a promising future for drug discovery.
In conclusion, the role of artificial intelligence in drug discovery is undeniably transformative, offering unprecedented opportunities to enhance the efficiency, accuracy, and scope of pharmaceutical research. As AI continues to evolve, its integration into drug development processes will likely lead to more innovative and effective treatments, ultimately improving patient outcomes and revolutionizing the healthcare landscape.
Advances in Nanotechnology for Targeted Drug Delivery
In recent years, the field of nanotechnology has emerged as a transformative force in the realm of drug and medicine development, offering unprecedented opportunities for targeted drug delivery. This innovative approach leverages the unique properties of nanoparticles to enhance the precision and efficacy of therapeutic interventions, thereby addressing some of the most pressing challenges in modern medicine. As researchers continue to explore the potential of nanotechnology, it becomes increasingly clear that these advancements hold the promise of revolutionizing the way we approach treatment for a myriad of diseases.
One of the primary advantages of using nanotechnology in drug delivery is its ability to improve the bioavailability of drugs. Traditional drug delivery methods often face significant obstacles, such as poor solubility and rapid degradation, which can limit the effectiveness of the treatment. Nanoparticles, however, can be engineered to encapsulate drugs, protecting them from premature degradation and enhancing their solubility. This encapsulation not only ensures that a higher concentration of the drug reaches the target site but also allows for controlled and sustained release, thereby reducing the frequency of dosing and minimizing side effects.
Moreover, the small size and customizable surface properties of nanoparticles enable them to navigate the complex biological environment of the human body with remarkable precision. By modifying the surface of these particles with specific ligands, researchers can design nanoparticles that selectively bind to target cells or tissues. This targeted approach is particularly beneficial in the treatment of cancer, where conventional therapies often harm healthy cells alongside malignant ones. Nanoparticles can be engineered to recognize and bind to cancer cells specifically, delivering cytotoxic agents directly to the tumor while sparing healthy tissue, thus reducing collateral damage and improving patient outcomes.
In addition to improving targeting capabilities, nanotechnology also facilitates the development of multifunctional drug delivery systems. These systems can be designed to perform multiple tasks simultaneously, such as imaging, diagnosis, and therapy. For instance, nanoparticles can be loaded with both therapeutic agents and imaging contrast materials, allowing clinicians to monitor the distribution and efficacy of the treatment in real-time. This integration of diagnostic and therapeutic functions, often referred to as “theranostics,” represents a significant leap forward in personalized medicine, enabling tailored treatment strategies that are responsive to the individual needs of patients.
Despite these promising advancements, the application of nanotechnology in drug delivery is not without its challenges. The complexity of designing nanoparticles that are both effective and safe for human use requires meticulous research and rigorous testing. Concerns regarding the long-term biocompatibility and potential toxicity of nanoparticles must be addressed to ensure that these innovative solutions do not pose unforeseen risks to patients. Furthermore, the regulatory landscape for nanomedicine is still evolving, necessitating the development of standardized guidelines to facilitate the translation of these technologies from the laboratory to the clinic.
In conclusion, the integration of nanotechnology into drug and medicine development represents a pioneering breakthrough with the potential to transform healthcare. By enhancing the precision and efficacy of drug delivery, nanotechnology offers a promising avenue for overcoming some of the most significant limitations of traditional therapies. As research in this field continues to advance, it is imperative that scientists, clinicians, and regulatory bodies work collaboratively to harness the full potential of these innovations, ensuring that they are both safe and accessible to those in need. Through such concerted efforts, the future of medicine may well be defined by the remarkable capabilities of nanotechnology.
The Future of mRNA Vaccines Beyond COVID-19
Messenger RNA (mRNA) vaccines have emerged as a groundbreaking innovation in the field of medicine, particularly highlighted by their pivotal role in combating the COVID-19 pandemic. However, the potential applications of mRNA technology extend far beyond the current global health crisis, promising to revolutionize the future of vaccine development and therapeutic interventions. As researchers continue to explore the versatility of mRNA, it becomes increasingly evident that this technology could address a myriad of diseases, offering new hope for conditions that have long eluded effective treatment.
To understand the future potential of mRNA vaccines, it is essential to first appreciate their underlying mechanism. Unlike traditional vaccines, which often use weakened or inactivated forms of a virus to elicit an immune response, mRNA vaccines work by instructing cells to produce a protein that triggers immunity. This approach not only accelerates the development process but also enhances the precision with which vaccines can be tailored to target specific pathogens. Consequently, the adaptability of mRNA technology positions it as a formidable tool in the fight against infectious diseases.
Beyond infectious diseases, mRNA vaccines hold promise in the realm of oncology. Cancer treatment has historically been challenged by the heterogeneity of tumors and the complexity of the immune system’s response. However, mRNA vaccines can be designed to encode tumor-specific antigens, thereby training the immune system to recognize and attack cancer cells. Early clinical trials have shown encouraging results, suggesting that mRNA-based cancer vaccines could become a cornerstone of personalized cancer therapy. This potential for customization underscores the transformative impact mRNA technology could have on cancer treatment paradigms.
Moreover, the flexibility of mRNA extends to addressing rare genetic disorders. By delivering mRNA that encodes functional proteins, researchers aim to correct genetic deficiencies at their source. This approach could offer a lifeline to patients with conditions that currently have limited or no treatment options. The ability to rapidly design and produce mRNA sequences tailored to individual genetic profiles exemplifies the precision medicine approach, which is increasingly becoming a focal point in modern healthcare.
In addition to therapeutic applications, mRNA technology is poised to enhance global health security. The rapid development and deployment of COVID-19 vaccines demonstrated the potential for mRNA platforms to respond swiftly to emerging infectious threats. This capability is crucial in an era where zoonotic diseases and pandemics pose significant risks to public health. By establishing robust mRNA vaccine infrastructure, the global community can be better prepared to mitigate future outbreaks, thereby safeguarding populations worldwide.
Despite these promising prospects, challenges remain in the widespread adoption of mRNA vaccines. Issues such as stability, delivery mechanisms, and manufacturing scalability must be addressed to fully realize the potential of this technology. Ongoing research and collaboration among scientists, industry leaders, and policymakers are essential to overcoming these hurdles and ensuring that mRNA vaccines can be effectively integrated into healthcare systems globally.
In conclusion, the future of mRNA vaccines extends far beyond their initial success in addressing COVID-19. With applications ranging from infectious diseases to cancer and genetic disorders, mRNA technology represents a paradigm shift in drug and medicine development. As research continues to advance, the potential for mRNA vaccines to transform healthcare and improve patient outcomes becomes increasingly apparent, heralding a new era of medical innovation.
Q&A
1. **What was the first antibiotic discovered, and who discovered it?**
– Penicillin was the first antibiotic discovered by Alexander Fleming in 1928.
2. **What is CRISPR-Cas9, and why is it significant in medicine?**
– CRISPR-Cas9 is a gene-editing technology that allows for precise modifications to DNA. It is significant because it holds potential for treating genetic disorders by correcting mutations at their source.
3. **What was the first vaccine ever developed, and who developed it?**
– The first vaccine was developed for smallpox by Edward Jenner in 1796.
4. **What is monoclonal antibody therapy, and what is its role in modern medicine?**
– Monoclonal antibody therapy involves using lab-created antibodies to target specific cells, such as cancer cells. It plays a crucial role in treating various diseases, including cancers and autoimmune disorders.
5. **What breakthrough drug was developed for the treatment of HIV/AIDS, and when was it introduced?**
– AZT (zidovudine) was the first breakthrough drug for HIV/AIDS treatment, introduced in 1987.
6. **What is the significance of mRNA technology in vaccine development?**
– mRNA technology allows for rapid development and production of vaccines, as demonstrated by the COVID-19 vaccines. It works by instructing cells to produce a protein that triggers an immune response.Pioneering breakthroughs in drug and medicine development have revolutionized healthcare, leading to significant improvements in patient outcomes and quality of life. Advances in genomics, biotechnology, and personalized medicine have enabled the creation of targeted therapies that are more effective and have fewer side effects. The integration of artificial intelligence and machine learning in drug discovery processes has accelerated the identification of potential drug candidates, reducing the time and cost associated with bringing new treatments to market. Additionally, innovations in drug delivery systems and regenerative medicine are opening new frontiers in treating previously intractable conditions. These breakthroughs not only enhance our understanding of disease mechanisms but also pave the way for more precise and individualized treatment approaches, ultimately transforming the landscape of modern medicine.
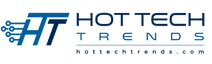