Nanotechnology in material science represents a transformative frontier that is reshaping the way materials are understood, designed, and utilized. By manipulating matter at the atomic and molecular scale, typically within the range of 1 to 100 nanometers, nanotechnology enables the creation of materials with unprecedented properties and functionalities. This field leverages the unique phenomena that occur at the nanoscale, such as quantum effects and increased surface area-to-volume ratios, to develop materials with enhanced strength, lighter weight, improved chemical reactivity, and superior electrical conductivity. These advancements are driving innovation across various industries, including electronics, medicine, energy, and environmental science, offering solutions that were previously unattainable with conventional materials. As research and development in nanotechnology continue to progress, the potential for groundbreaking applications in material science expands, promising to revolutionize technology and improve quality of life.
Advances In Nanocomposite Materials
Nanotechnology has revolutionized various fields, and its impact on material science is particularly profound. The development of nanocomposite materials represents a significant advancement, offering enhanced properties and functionalities that were previously unattainable with conventional materials. These materials, which incorporate nanoparticles into a matrix of standard materials, have opened new avenues for innovation and application across diverse industries.
One of the most remarkable aspects of nanocomposite materials is their ability to improve mechanical properties. By integrating nanoparticles such as carbon nanotubes, graphene, or silica into polymers, researchers have achieved significant enhancements in strength, toughness, and durability. This improvement is primarily due to the high surface area-to-volume ratio of nanoparticles, which allows for better stress distribution and load transfer within the composite. Consequently, these materials are finding applications in sectors ranging from aerospace to automotive, where lightweight yet strong materials are crucial.
In addition to mechanical enhancements, nanocomposite materials also exhibit superior thermal and electrical properties. For instance, the inclusion of metallic or carbon-based nanoparticles can significantly increase the thermal conductivity of a polymer matrix. This property is particularly beneficial in electronics, where efficient heat dissipation is essential to prevent overheating and ensure device longevity. Similarly, the electrical conductivity of polymers can be dramatically improved by incorporating conductive nanoparticles, paving the way for flexible electronics and advanced sensors.
Moreover, the versatility of nanocomposite materials extends to their optical properties. By manipulating the size, shape, and distribution of nanoparticles within a matrix, researchers can tailor the optical characteristics of the material. This capability is instrumental in developing advanced coatings and films with specific light absorption, reflection, or transmission properties. Such materials are invaluable in applications like solar energy harvesting, where maximizing light absorption is critical for efficiency.
The environmental impact of materials is an increasingly important consideration, and nanocomposite materials offer promising solutions in this regard. For example, the incorporation of nanoparticles can enhance the barrier properties of packaging materials, reducing the need for additional layers and thus minimizing waste. Furthermore, certain nanocomposites are being developed with self-healing capabilities, which can extend the lifespan of products and reduce the frequency of replacements. This not only conserves resources but also decreases the environmental footprint associated with manufacturing and disposal.
Despite these advancements, challenges remain in the widespread adoption of nanocomposite materials. One significant hurdle is the scalability of production processes. While laboratory-scale synthesis of nanocomposites has been successful, translating these methods to industrial scales without compromising quality or cost-effectiveness is a complex task. Additionally, there are concerns regarding the health and environmental implications of nanoparticles, necessitating thorough research and regulation to ensure safe use.
In conclusion, the advances in nanocomposite materials underscore the transformative potential of nanotechnology in material science. By enhancing mechanical, thermal, electrical, and optical properties, these materials are poised to drive innovation across multiple industries. However, addressing the challenges of scalability and safety will be crucial to fully realizing their benefits. As research continues to evolve, the future of nanocomposite materials looks promising, with the potential to significantly impact both technology and sustainability.
Nanotechnology In Energy Storage Solutions
Nanotechnology has emerged as a transformative force in various fields, and its impact on energy storage solutions is particularly noteworthy. As the demand for efficient and sustainable energy storage systems grows, nanotechnology offers promising advancements that could revolutionize the way energy is stored and utilized. By manipulating materials at the nanoscale, scientists and engineers are developing innovative solutions that enhance the performance, capacity, and longevity of energy storage devices.
One of the most significant contributions of nanotechnology to energy storage is the development of advanced batteries. Traditional batteries, such as lithium-ion, have limitations in terms of energy density and charging speed. However, by incorporating nanomaterials, researchers have been able to address these challenges. For instance, the use of nanostructured electrodes can significantly increase the surface area available for chemical reactions, thereby enhancing the battery’s capacity and efficiency. Moreover, nanotechnology enables the creation of more stable and durable materials, which can lead to longer battery life and improved safety.
In addition to batteries, nanotechnology is also making strides in the field of supercapacitors. Supercapacitors are known for their ability to charge and discharge rapidly, making them ideal for applications requiring quick bursts of energy. By utilizing nanomaterials, such as graphene and carbon nanotubes, researchers have been able to improve the energy density of supercapacitors, bringing them closer to the storage capacity of traditional batteries. This advancement not only enhances the performance of supercapacitors but also broadens their potential applications, from consumer electronics to electric vehicles.
Furthermore, nanotechnology is playing a crucial role in the development of next-generation energy storage systems, such as solid-state batteries. Solid-state batteries, which use a solid electrolyte instead of a liquid one, offer several advantages, including higher energy density and improved safety. Nanotechnology facilitates the design of solid electrolytes with superior ionic conductivity and mechanical properties, overcoming some of the key challenges associated with solid-state battery technology. As a result, these batteries are becoming increasingly viable for commercial use, promising to deliver more efficient and reliable energy storage solutions.
Moreover, the integration of nanotechnology in energy storage is not limited to enhancing existing technologies. It also paves the way for entirely new approaches, such as the development of flexible and wearable energy storage devices. By leveraging the unique properties of nanomaterials, researchers are creating thin, lightweight, and flexible batteries and supercapacitors that can be integrated into textiles and other materials. This innovation opens up exciting possibilities for powering wearable electronics and other portable devices, providing a seamless and convenient energy solution.
In conclusion, nanotechnology is poised to play a pivotal role in the evolution of energy storage solutions. By enabling the development of more efficient, durable, and versatile storage devices, it addresses some of the most pressing challenges in the energy sector. As research and development in this field continue to advance, the integration of nanotechnology in energy storage systems holds the potential to significantly impact various industries, from consumer electronics to renewable energy. Ultimately, these advancements could lead to a more sustainable and energy-efficient future, underscoring the importance of continued investment and exploration in nanotechnology for material science.
Nanostructured Coatings For Corrosion Resistance
Nanotechnology has revolutionized various fields, and its impact on material science is particularly profound. One of the most promising applications of nanotechnology in this domain is the development of nanostructured coatings for corrosion resistance. Corrosion, a natural process that deteriorates materials, especially metals, poses significant challenges across numerous industries, including construction, transportation, and energy. The economic implications of corrosion are substantial, with billions of dollars spent annually on prevention and maintenance. Consequently, the quest for effective corrosion-resistant solutions is of paramount importance.
Nanostructured coatings offer a novel approach to combating corrosion by leveraging the unique properties of materials at the nanoscale. These coatings are engineered with nanoparticles or nanolayers that provide superior protection compared to traditional coatings. The enhanced performance of nanostructured coatings can be attributed to several factors, including their high surface area-to-volume ratio, which allows for better adhesion and coverage on the substrate. Additionally, the nanoscale features can create a more uniform and dense barrier, effectively blocking corrosive agents such as water, oxygen, and salts from reaching the underlying material.
Moreover, the versatility of nanostructured coatings is noteworthy. By manipulating the composition and structure of the nanoparticles, researchers can tailor the coatings to meet specific requirements. For instance, incorporating nanoparticles of materials like titanium dioxide or zinc oxide can impart self-healing properties to the coatings. When the coating is damaged, these nanoparticles can react with environmental moisture to form protective layers that seal the breach, thereby extending the lifespan of the coating and the substrate it protects.
Furthermore, the development of nanostructured coatings is not limited to metallic substrates. These coatings can also be applied to non-metallic materials, such as polymers and ceramics, broadening their applicability across different sectors. This adaptability is crucial as industries seek to protect a wide range of materials from corrosion, ensuring the longevity and reliability of their products.
In addition to their protective capabilities, nanostructured coatings can also enhance the aesthetic and functional properties of materials. For example, they can be engineered to provide anti-reflective, anti-fogging, or self-cleaning surfaces, adding value beyond corrosion resistance. This multifunctionality is particularly advantageous in industries where both performance and appearance are critical, such as in automotive and consumer electronics.
Despite the promising potential of nanostructured coatings, challenges remain in their widespread adoption. The production and application processes of these coatings can be complex and costly, necessitating further research and development to optimize their scalability and cost-effectiveness. Additionally, the long-term environmental and health impacts of nanoparticles are still under investigation, requiring careful consideration and regulation to ensure safe implementation.
In conclusion, nanostructured coatings represent a significant advancement in the fight against corrosion, offering enhanced protection and versatility compared to traditional methods. As research continues to address the challenges associated with their use, these coatings are poised to become an integral part of material science, providing durable and multifunctional solutions across various industries. The ongoing exploration of nanotechnology’s potential in this field promises to yield innovative approaches that will redefine how we protect and preserve materials in the future.
Nanomaterials In Biomedical Applications
Nanotechnology has emerged as a transformative force in material science, particularly in the realm of biomedical applications. The integration of nanomaterials into medical technologies has opened new avenues for diagnosis, treatment, and prevention of diseases, offering unprecedented precision and efficiency. As researchers continue to explore the potential of these materials, it becomes increasingly clear that nanotechnology holds the key to revolutionizing healthcare.
One of the most promising applications of nanomaterials in biomedicine is in drug delivery systems. Traditional drug delivery methods often face challenges such as poor solubility, limited bioavailability, and non-specific targeting, which can lead to suboptimal therapeutic outcomes and adverse side effects. Nanomaterials, however, offer a solution to these issues by enabling the design of drug delivery systems that can precisely target diseased cells while minimizing damage to healthy tissues. For instance, nanoparticles can be engineered to carry therapeutic agents directly to cancer cells, thereby enhancing the efficacy of chemotherapy while reducing its toxic side effects. This targeted approach not only improves patient outcomes but also paves the way for personalized medicine.
In addition to drug delivery, nanomaterials are also making significant strides in the field of diagnostics. The ability to detect diseases at an early stage is crucial for effective treatment, and nanotechnology offers tools that can significantly enhance diagnostic capabilities. Nanoscale sensors, for example, can detect biomarkers associated with various diseases at extremely low concentrations, allowing for early and accurate diagnosis. These sensors can be integrated into portable devices, making it possible to conduct point-of-care testing and obtain rapid results. This advancement is particularly beneficial in resource-limited settings, where access to traditional diagnostic facilities may be limited.
Furthermore, nanomaterials are being utilized in the development of advanced imaging techniques. Imaging is a critical component of medical diagnostics, and the incorporation of nanotechnology has led to the creation of contrast agents that improve the resolution and specificity of imaging modalities such as MRI and CT scans. These nanomaterial-based contrast agents can provide detailed images of biological structures, facilitating better diagnosis and monitoring of diseases. Moreover, the use of nanotechnology in imaging extends beyond diagnostics; it also plays a role in guiding surgical procedures and monitoring the progress of treatments.
Beyond diagnostics and drug delivery, nanomaterials are also contributing to the development of regenerative medicine. The ability to repair or replace damaged tissues and organs is a long-standing goal in medicine, and nanotechnology is helping to bring this vision closer to reality. Nanomaterials can be used to create scaffolds that mimic the extracellular matrix, providing a supportive environment for cell growth and tissue regeneration. These scaffolds can be tailored to promote the regeneration of specific tissues, such as bone, cartilage, or skin, offering new hope for patients with injuries or degenerative diseases.
In conclusion, the application of nanomaterials in biomedical fields is a rapidly evolving area that holds immense potential for improving healthcare outcomes. From enhancing drug delivery and diagnostics to advancing imaging techniques and regenerative medicine, nanotechnology is poised to address some of the most pressing challenges in medicine today. As research continues to advance, it is likely that the integration of nanomaterials into medical practice will lead to more effective, personalized, and accessible healthcare solutions, ultimately transforming the landscape of modern medicine.
Environmental Impact Of Nanomaterials
Nanotechnology has emerged as a transformative force in material science, offering unprecedented opportunities to enhance material properties and functionalities. However, as the application of nanomaterials expands, it is crucial to consider their environmental impact. The unique properties of nanomaterials, which make them so valuable in various applications, also pose distinct challenges when it comes to their interaction with the environment. Understanding these impacts is essential for developing sustainable practices in the field of nanotechnology.
To begin with, the environmental impact of nanomaterials is largely influenced by their size, shape, surface area, and chemical composition. These factors determine how nanomaterials interact with biological systems and ecosystems. For instance, due to their small size, nanomaterials can easily penetrate biological membranes, potentially leading to bioaccumulation and toxicity in living organisms. This raises concerns about the long-term effects of nanomaterials on wildlife and human health, particularly when they are released into the environment through industrial processes or consumer products.
Moreover, the production and disposal of nanomaterials present additional environmental challenges. The manufacturing processes often involve the use of hazardous chemicals and generate waste that can be difficult to manage. As nanomaterials are integrated into products, their lifecycle must be carefully considered to prevent environmental contamination. For example, when products containing nanomaterials reach the end of their life, they may release nanoparticles into the environment, where they can persist and potentially cause harm.
In addition to these direct impacts, nanomaterials can also influence environmental processes in more subtle ways. For example, they can affect the behavior of pollutants in the environment. Some nanomaterials have been shown to enhance the degradation of organic pollutants, offering potential benefits for environmental remediation. However, they can also facilitate the transport of pollutants, increasing their mobility and potentially leading to wider distribution in the environment. This dual role underscores the complexity of assessing the environmental impact of nanomaterials.
Furthermore, the environmental impact of nanomaterials is not uniform and can vary significantly depending on the specific type of nanomaterial and its application. For instance, carbon-based nanomaterials, such as carbon nanotubes and graphene, have different environmental interactions compared to metal-based nanomaterials like silver or titanium dioxide nanoparticles. Each type of nanomaterial requires a tailored approach to assess its environmental risks and benefits.
To address these challenges, researchers and policymakers are working to develop frameworks for the safe and sustainable use of nanomaterials. This includes establishing guidelines for the assessment of environmental risks, promoting the development of greener synthesis methods, and encouraging the design of nanomaterials that are less likely to cause harm. Additionally, there is a growing emphasis on life cycle assessment to evaluate the environmental impact of nanomaterials from production to disposal.
In conclusion, while nanotechnology holds great promise for advancing material science, it is imperative to carefully consider the environmental impact of nanomaterials. By understanding and mitigating these impacts, we can harness the benefits of nanotechnology while safeguarding the environment. As research in this field continues to evolve, it will be essential to balance innovation with responsibility, ensuring that the development of nanomaterials contributes to a sustainable future.
Nanotechnology In Sustainable Construction Materials
Nanotechnology has emerged as a transformative force in various fields, and its application in material science, particularly in sustainable construction materials, is garnering significant attention. As the world grapples with the challenges of climate change and resource depletion, the construction industry is under increasing pressure to adopt more sustainable practices. Nanotechnology offers promising solutions by enhancing the properties of construction materials, thereby improving their performance and sustainability.
One of the primary ways nanotechnology contributes to sustainable construction is through the development of high-performance concrete. Traditional concrete, while widely used, has significant environmental drawbacks, including high carbon emissions during production. By incorporating nanoparticles such as nano-silica, nano-titanium dioxide, and carbon nanotubes, researchers have been able to improve the mechanical properties of concrete. These nanoparticles enhance the material’s strength, durability, and resistance to environmental factors, thereby extending the lifespan of concrete structures and reducing the need for frequent repairs and replacements. Consequently, this leads to a decrease in the consumption of raw materials and energy, contributing to a more sustainable construction process.
In addition to improving concrete, nanotechnology is also being utilized to develop advanced insulation materials. Energy efficiency is a critical aspect of sustainable construction, and effective insulation plays a vital role in reducing energy consumption in buildings. Aerogels, which are nanostructured materials, have emerged as a superior insulation option due to their low thermal conductivity and lightweight nature. These materials provide excellent thermal insulation while occupying minimal space, making them ideal for use in both new constructions and retrofitting existing buildings. By reducing the energy required for heating and cooling, aerogels contribute significantly to lowering the carbon footprint of buildings.
Moreover, nanotechnology is paving the way for the development of self-cleaning and air-purifying surfaces. Coatings containing nanoparticles such as titanium dioxide can be applied to building exteriors, enabling them to break down pollutants and organic matter when exposed to sunlight. This photocatalytic process not only keeps surfaces clean but also improves air quality by reducing harmful emissions. Such innovations are particularly beneficial in urban areas, where pollution levels are high, and maintaining clean building facades is challenging.
Furthermore, the integration of nanotechnology in construction materials extends to the development of smart materials that can respond to environmental changes. For instance, shape-memory alloys and polymers can be used in structures that require adaptability to varying conditions, such as bridges and high-rise buildings. These materials can change their properties in response to temperature fluctuations or mechanical stress, enhancing the safety and resilience of structures. By incorporating such smart materials, the construction industry can create buildings and infrastructure that are not only more sustainable but also more responsive to the dynamic challenges posed by climate change.
In conclusion, the application of nanotechnology in sustainable construction materials holds immense potential for revolutionizing the construction industry. By enhancing the properties of traditional materials and introducing innovative solutions, nanotechnology contributes to the development of more durable, energy-efficient, and environmentally friendly construction practices. As research and development in this field continue to advance, it is anticipated that nanotechnology will play an increasingly pivotal role in shaping the future of sustainable construction, ultimately leading to a more sustainable built environment.
Q&A
1. **What is nanotechnology in material science?**
Nanotechnology in material science involves manipulating materials at the nanoscale (1 to 100 nanometers) to enhance their properties, such as strength, flexibility, conductivity, and reactivity, leading to innovative applications across various industries.
2. **How does nanotechnology improve material properties?**
Nanotechnology improves material properties by altering the structure at the atomic or molecular level, which can result in increased strength, lighter weight, improved thermal and electrical conductivity, and enhanced chemical reactivity.
3. **What are some applications of nanotechnology in material science?**
Applications include the development of stronger and lighter materials for aerospace and automotive industries, improved drug delivery systems in medicine, enhanced energy storage in batteries, and the creation of self-cleaning and anti-reflective coatings.
4. **What are carbon nanotubes and their significance in material science?**
Carbon nanotubes are cylindrical nanostructures with exceptional strength, electrical conductivity, and thermal properties, making them significant for developing advanced composites, electronics, and energy storage devices.
5. **What role does nanotechnology play in sustainable materials?**
Nanotechnology contributes to sustainable materials by enabling the development of more efficient energy storage systems, reducing material waste through enhanced recyclability, and creating materials that require less energy to produce and maintain.
6. **What are the challenges of using nanotechnology in material science?**
Challenges include potential health and environmental risks, high production costs, difficulties in large-scale manufacturing, and the need for standardized regulations and testing methods to ensure safety and efficacy.Nanotechnology in material science represents a transformative frontier that is reshaping the way materials are designed, synthesized, and utilized. By manipulating matter at the nanoscale, scientists and engineers can enhance material properties such as strength, flexibility, conductivity, and reactivity, leading to innovations across various industries. This technology enables the development of advanced materials with unprecedented capabilities, such as self-healing composites, highly efficient catalysts, and superior electronic components. As research progresses, nanotechnology holds the promise of addressing critical global challenges, including energy sustainability, environmental remediation, and healthcare advancements. However, it also necessitates careful consideration of potential risks and ethical implications. Overall, nanotechnology in material science is poised to drive significant technological progress, offering solutions that were previously unimaginable.
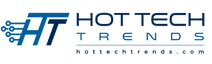