Biotechnology has emerged as a transformative force in healthcare, driving significant advancements that are reshaping the landscape of medical science and patient care. By harnessing the power of biological systems, organisms, and cellular processes, biotechnology is enabling the development of innovative therapies, diagnostic tools, and personalized medicine approaches. These advancements are not only enhancing our understanding of complex diseases but also paving the way for more effective and targeted treatments. From the engineering of novel biopharmaceuticals and the application of CRISPR gene-editing technology to the creation of sophisticated diagnostic assays and regenerative medicine techniques, biotechnology is at the forefront of a healthcare revolution. This dynamic field is addressing some of the most pressing medical challenges, offering new hope for patients and promising to improve health outcomes on a global scale.
Gene Editing And CRISPR Technology
Biotechnology has revolutionized the healthcare landscape, with gene editing and CRISPR technology standing at the forefront of this transformation. These advancements have opened new avenues for treating genetic disorders, enhancing our understanding of complex diseases, and potentially eradicating hereditary conditions. As we delve into the intricacies of gene editing, it is essential to appreciate the profound impact CRISPR technology has had on the field.
Gene editing, a method that allows scientists to alter an organism’s DNA, has been a subject of research for decades. However, the advent of CRISPR-Cas9 technology has significantly accelerated progress in this domain. CRISPR, which stands for Clustered Regularly Interspaced Short Palindromic Repeats, is a revolutionary tool that enables precise, directed changes to genomic DNA. The simplicity, efficiency, and cost-effectiveness of CRISPR have made it a preferred method for gene editing, surpassing previous techniques such as zinc finger nucleases and TALENs.
The potential applications of CRISPR technology in healthcare are vast and varied. One of the most promising areas is the treatment of genetic disorders. Diseases such as cystic fibrosis, sickle cell anemia, and muscular dystrophy, which are caused by specific genetic mutations, could potentially be corrected using CRISPR. By directly targeting and repairing the faulty genes responsible for these conditions, CRISPR offers hope for permanent cures rather than merely managing symptoms.
Moreover, CRISPR technology is not limited to monogenic disorders. It also holds promise for complex diseases like cancer. Researchers are exploring ways to use CRISPR to modify immune cells, enabling them to better recognize and attack cancer cells. This approach, known as immunotherapy, could lead to more effective and personalized cancer treatments. Additionally, CRISPR can be used to create more accurate disease models, facilitating the development of new drugs and therapies.
Despite its potential, the use of CRISPR technology in healthcare is not without challenges. Ethical considerations are paramount, particularly when it comes to germline editing, which involves making changes that can be passed on to future generations. The possibility of unintended off-target effects, where CRISPR inadvertently alters genes other than the intended target, also raises safety concerns. As such, rigorous testing and regulatory oversight are essential to ensure that CRISPR-based therapies are both safe and effective.
Furthermore, the accessibility of CRISPR technology poses questions about equity in healthcare. While the technology itself is relatively inexpensive, the development and delivery of CRISPR-based treatments may not be affordable for all. Ensuring that these advancements benefit a broad spectrum of patients, regardless of socioeconomic status, is a challenge that the healthcare community must address.
In conclusion, gene editing and CRISPR technology represent a significant leap forward in biotechnology, with the potential to transform healthcare as we know it. By offering new solutions for genetic disorders and complex diseases, CRISPR holds the promise of more effective, personalized treatments. However, as we continue to explore its possibilities, it is crucial to navigate the ethical, safety, and accessibility challenges that accompany these advancements. Through careful consideration and responsible application, CRISPR technology can pave the way for a new era in medicine, improving health outcomes for individuals worldwide.
Personalized Medicine And Genomics
Biotechnology has revolutionized the healthcare landscape, particularly through the advancements in personalized medicine and genomics. These fields have transformed the traditional one-size-fits-all approach to treatment, offering a more tailored strategy that considers individual genetic profiles. Personalized medicine, also known as precision medicine, leverages genomic information to guide decisions made in regard to the prevention, diagnosis, and treatment of diseases. This approach not only enhances the efficacy of treatments but also minimizes adverse effects, thereby improving patient outcomes.
The integration of genomics into healthcare has been facilitated by the rapid advancements in sequencing technologies. The cost of sequencing a human genome has plummeted since the completion of the Human Genome Project, making it more accessible for clinical use. This affordability has enabled healthcare providers to incorporate genetic testing into routine medical care, allowing for the identification of genetic predispositions to certain diseases. Consequently, individuals can receive more accurate risk assessments and engage in proactive health management strategies.
Moreover, personalized medicine has shown significant promise in the field of oncology. Cancer treatment has traditionally been challenging due to the heterogeneity of tumors, which often leads to variable responses to standard therapies. However, by analyzing the genetic mutations specific to a patient’s tumor, oncologists can now select targeted therapies that are more likely to be effective. This approach not only increases the likelihood of treatment success but also reduces the exposure to potentially harmful side effects associated with conventional chemotherapy.
In addition to oncology, personalized medicine is making strides in the management of chronic diseases such as cardiovascular disorders and diabetes. For instance, pharmacogenomics, a subset of personalized medicine, examines how genes affect a person’s response to drugs. This knowledge allows for the customization of drug therapies, ensuring that patients receive medications that are most compatible with their genetic makeup. As a result, healthcare providers can optimize drug efficacy and minimize the risk of adverse drug reactions, which are a significant cause of morbidity and mortality.
Furthermore, the integration of genomics into healthcare is fostering the development of novel therapeutic approaches, such as gene therapy and CRISPR-based gene editing. These technologies hold the potential to correct genetic defects at their source, offering the possibility of curing genetic disorders that were previously deemed untreatable. While these therapies are still in the experimental stages, their successful implementation could mark a paradigm shift in the treatment of genetic diseases.
Despite the promising advancements, the implementation of personalized medicine and genomics in healthcare is not without challenges. Ethical considerations, such as patient privacy and data security, are paramount, given the sensitive nature of genetic information. Additionally, there is a need for healthcare professionals to receive adequate training in genomics to effectively interpret and apply genetic data in clinical practice. Addressing these challenges will be crucial to fully realizing the potential of personalized medicine.
In conclusion, biotechnology advances in personalized medicine and genomics are paving the way for a more individualized approach to healthcare. By harnessing the power of genetic information, these fields are enhancing the precision of medical interventions, improving patient outcomes, and opening new avenues for the treatment of complex diseases. As research continues to evolve, the integration of these technologies into mainstream healthcare is likely to become increasingly prevalent, heralding a new era of medicine that is as unique as the individuals it serves.
Bioprinting And Tissue Engineering
Biotechnology has been at the forefront of transformative changes in healthcare, with bioprinting and tissue engineering emerging as pivotal areas of innovation. These technologies hold the promise of revolutionizing medical treatments by enabling the creation of complex biological structures that can mimic natural tissues. As the field progresses, it is essential to understand the intricacies and potential applications of these groundbreaking technologies.
Bioprinting, a subset of additive manufacturing, involves the precise layer-by-layer deposition of bioinks to create three-dimensional structures. These bioinks are typically composed of living cells, biomaterials, and growth factors, which together form the building blocks of tissues. The process begins with the design of a digital model, often derived from medical imaging data, which guides the printer in constructing the desired tissue. This technology offers unparalleled precision and customization, allowing for the creation of patient-specific tissues that can potentially reduce the risk of rejection in transplants.
Transitioning to tissue engineering, this field focuses on the development of biological substitutes that can restore, maintain, or improve tissue function. It combines principles from engineering and life sciences to fabricate scaffolds that support cell growth and tissue development. These scaffolds, often made from biodegradable materials, provide a temporary structure that cells can adhere to and proliferate within, eventually forming functional tissue. The integration of bioprinting with tissue engineering has accelerated advancements, enabling the production of more complex and viable tissue constructs.
The potential applications of bioprinting and tissue engineering in healthcare are vast and varied. One of the most promising areas is in regenerative medicine, where these technologies can be used to repair or replace damaged tissues and organs. For instance, bioprinted skin grafts are being developed to treat burn victims, offering a more effective and personalized solution compared to traditional methods. Similarly, researchers are exploring the creation of bioprinted cartilage for joint repair, which could significantly improve the quality of life for individuals suffering from osteoarthritis.
Moreover, these technologies are making significant strides in drug development and testing. Bioprinted tissues can serve as more accurate models for studying disease progression and drug responses, reducing the reliance on animal testing and improving the predictability of clinical outcomes. This approach not only accelerates the drug discovery process but also enhances the safety and efficacy of new treatments.
Despite the remarkable progress, several challenges remain in the widespread adoption of bioprinting and tissue engineering. Technical hurdles, such as ensuring the vascularization and long-term viability of printed tissues, need to be addressed. Additionally, regulatory frameworks must evolve to accommodate these novel therapies, ensuring their safety and efficacy for clinical use. Ethical considerations, particularly concerning the use of human cells and genetic materials, also require careful deliberation.
In conclusion, bioprinting and tissue engineering represent a paradigm shift in healthcare, offering innovative solutions to some of the most pressing medical challenges. As research and development continue to advance, these technologies have the potential to transform the landscape of medicine, providing new hope for patients worldwide. By addressing the existing challenges and fostering collaboration across disciplines, the full potential of bioprinting and tissue engineering can be realized, paving the way for a new era of personalized and regenerative healthcare.
AI And Machine Learning In Drug Discovery
Biotechnology has been at the forefront of transformative changes in healthcare, and one of the most promising areas of advancement is the integration of artificial intelligence (AI) and machine learning in drug discovery. This innovative approach is revolutionizing the way new drugs are developed, offering the potential to significantly reduce the time and cost associated with bringing new therapies to market. As the pharmaceutical industry grapples with the challenges of high failure rates and escalating research and development costs, AI and machine learning present a compelling solution.
Traditionally, drug discovery has been a laborious and time-consuming process, often taking over a decade from initial research to market approval. However, AI and machine learning algorithms can analyze vast datasets with unprecedented speed and accuracy, identifying potential drug candidates much more efficiently than conventional methods. By leveraging these technologies, researchers can sift through millions of chemical compounds to pinpoint those most likely to yield effective treatments. This capability not only accelerates the initial stages of drug discovery but also enhances the precision of target identification, thereby increasing the likelihood of success in subsequent clinical trials.
Moreover, AI and machine learning facilitate the development of personalized medicine, a burgeoning field that tailors treatments to individual patients based on their genetic makeup. By analyzing genetic data, these technologies can predict how different patients will respond to specific drugs, enabling the design of more effective and personalized treatment regimens. This approach not only improves patient outcomes but also minimizes adverse effects, as treatments can be customized to align with each patient’s unique genetic profile.
In addition to identifying new drug candidates, AI and machine learning are instrumental in optimizing existing drugs. Through the analysis of clinical trial data and real-world evidence, these technologies can uncover new therapeutic uses for existing medications, a process known as drug repurposing. This strategy is particularly valuable as it allows for the rapid deployment of treatments for emerging diseases, leveraging the safety profiles of drugs that have already been approved for other indications.
Furthermore, AI-driven platforms are enhancing the efficiency of clinical trials, a critical phase in the drug development process. By predicting patient responses and identifying optimal patient populations, these technologies can streamline trial design and execution, reducing both time and cost. This capability is especially crucial in the context of rare diseases, where patient populations are limited, and traditional trial methods may be impractical.
Despite the promising potential of AI and machine learning in drug discovery, challenges remain. The integration of these technologies requires significant investment in infrastructure and expertise, and there are concerns regarding data privacy and security. Additionally, the regulatory landscape must evolve to accommodate the unique aspects of AI-driven drug development, ensuring that these innovations meet rigorous safety and efficacy standards.
In conclusion, the application of AI and machine learning in drug discovery represents a paradigm shift in biotechnology, offering the promise of more efficient, cost-effective, and personalized healthcare solutions. As these technologies continue to advance, they hold the potential to transform the pharmaceutical industry, ultimately improving patient outcomes and addressing unmet medical needs. The journey towards fully realizing this potential is ongoing, but the progress made thus far underscores the transformative impact of AI and machine learning in the realm of drug discovery.
Nanotechnology In Targeted Drug Delivery
Nanotechnology has emerged as a transformative force in the field of biotechnology, particularly in the realm of targeted drug delivery. This innovative approach leverages the unique properties of nanoparticles to enhance the precision and efficacy of therapeutic interventions. As the healthcare industry continues to evolve, the integration of nanotechnology into drug delivery systems promises to revolutionize the way diseases are treated, offering new hope for patients with conditions that have been challenging to manage with traditional therapies.
At the core of nanotechnology’s potential in targeted drug delivery is its ability to manipulate materials at the molecular and atomic levels. This capability allows for the design of nanoparticles that can carry drugs directly to specific cells or tissues, minimizing the impact on healthy cells and reducing side effects. For instance, in cancer treatment, where conventional chemotherapy often affects both cancerous and healthy cells, nanotechnology can be employed to deliver chemotherapeutic agents directly to tumor sites. This targeted approach not only enhances the effectiveness of the treatment but also significantly reduces the adverse effects typically associated with chemotherapy.
Moreover, the versatility of nanoparticles enables the development of multifunctional drug delivery systems. These systems can be engineered to respond to specific stimuli, such as changes in pH or temperature, which are often indicative of disease states. By incorporating these responsive elements, nanoparticles can release their therapeutic payloads in a controlled manner, ensuring that drugs are delivered at the optimal time and location within the body. This level of precision is particularly beneficial in treating diseases with complex pathophysiologies, such as autoimmune disorders and neurodegenerative diseases, where traditional drug delivery methods may fall short.
In addition to improving the targeting and release of drugs, nanotechnology also offers the potential to enhance the solubility and stability of therapeutic agents. Many drugs, particularly those that are hydrophobic, face challenges in terms of bioavailability and degradation before reaching their intended targets. Nanoparticles can be designed to encapsulate these drugs, protecting them from premature degradation and facilitating their transport through the body. This encapsulation not only improves the pharmacokinetic profiles of drugs but also allows for the use of compounds that were previously deemed unsuitable for therapeutic applications due to their poor solubility.
Furthermore, the integration of nanotechnology in drug delivery systems is paving the way for personalized medicine. By tailoring nanoparticles to the specific genetic and molecular profiles of individual patients, healthcare providers can develop customized treatment regimens that are more effective and have fewer side effects. This personalized approach is particularly promising in the treatment of genetic disorders and cancers, where the heterogeneity of the disease can significantly impact treatment outcomes.
Despite the promising advancements, the application of nanotechnology in targeted drug delivery is not without challenges. Regulatory hurdles, potential toxicity, and the complexity of manufacturing processes are some of the obstacles that need to be addressed to fully realize the potential of this technology. Nevertheless, ongoing research and development efforts continue to push the boundaries of what is possible, bringing us closer to a future where nanotechnology plays a central role in the delivery of safe and effective therapies.
In conclusion, nanotechnology represents a significant advancement in the field of biotechnology, offering innovative solutions for targeted drug delivery. By enhancing the precision, efficacy, and personalization of therapeutic interventions, this technology holds the promise of transforming healthcare and improving patient outcomes across a wide range of diseases. As research progresses, it is anticipated that nanotechnology will become an integral component of modern medicine, ushering in a new era of treatment possibilities.
Synthetic Biology And Vaccine Development
Biotechnology has revolutionized various sectors, with healthcare being one of the most significantly impacted. Among the numerous advancements, synthetic biology stands out as a transformative force, particularly in the realm of vaccine development. This innovative field combines principles from biology and engineering to design and construct new biological parts, devices, and systems. As a result, it has opened new avenues for creating vaccines that are not only more effective but also faster to develop and produce.
One of the primary advantages of synthetic biology in vaccine development is its ability to streamline the production process. Traditional vaccine development can be a lengthy and complex procedure, often taking years to bring a new vaccine to market. However, synthetic biology allows for the rapid synthesis of genetic material, enabling researchers to quickly design and test vaccine candidates. This capability was notably demonstrated during the COVID-19 pandemic, where synthetic biology played a crucial role in the rapid development of mRNA vaccines. By using synthetic RNA sequences, scientists were able to create vaccines that effectively instructed cells to produce viral proteins, thereby eliciting an immune response without the need for live virus cultures.
Moreover, synthetic biology facilitates the customization of vaccines to target specific pathogens more precisely. This precision is achieved through the design of synthetic antigens that mimic the structure of pathogens, allowing the immune system to recognize and combat them more effectively. Such targeted approaches not only enhance the efficacy of vaccines but also reduce the risk of adverse reactions, as they can be tailored to minimize unnecessary immune responses. Furthermore, synthetic biology enables the development of multivalent vaccines, which can protect against multiple strains of a virus or even different pathogens simultaneously. This is particularly beneficial in combating diseases with high mutation rates, such as influenza, where traditional vaccines often struggle to keep pace with evolving viral strains.
In addition to improving the speed and specificity of vaccine development, synthetic biology also offers solutions to some of the logistical challenges associated with vaccine distribution. For instance, synthetic biology techniques can be used to create vaccines that are more stable at room temperature, reducing the need for cold chain storage and making them more accessible in regions with limited infrastructure. This is a significant advancement, as it addresses one of the major barriers to global vaccination efforts, particularly in low- and middle-income countries.
Furthermore, the integration of synthetic biology with other biotechnological tools, such as CRISPR and bioinformatics, is paving the way for even more sophisticated vaccine designs. These technologies allow for the precise editing of genetic material and the analysis of vast amounts of biological data, respectively, enabling researchers to identify novel targets for vaccine development and optimize vaccine formulations. As these technologies continue to evolve, they hold the promise of further enhancing the effectiveness and accessibility of vaccines worldwide.
In conclusion, synthetic biology represents a paradigm shift in vaccine development, offering unprecedented opportunities to improve the speed, precision, and distribution of vaccines. As the field continues to advance, it is poised to play an increasingly vital role in addressing current and future public health challenges. By harnessing the power of synthetic biology, the healthcare industry can develop more effective vaccines, ultimately leading to better health outcomes and a more resilient global population.
Q&A
1. **What is CRISPR-Cas9 and how is it used in healthcare?**
CRISPR-Cas9 is a gene-editing technology that allows for precise modifications to DNA. In healthcare, it is used for developing treatments for genetic disorders by correcting mutations, potentially curing diseases like cystic fibrosis and sickle cell anemia.
2. **How are monoclonal antibodies utilized in modern medicine?**
Monoclonal antibodies are lab-produced molecules engineered to serve as substitute antibodies. They can restore, enhance, or mimic the immune system’s attack on cancer cells, making them effective in treating various cancers and autoimmune diseases.
3. **What role does biotechnology play in vaccine development?**
Biotechnology enables the rapid development of vaccines through techniques like recombinant DNA technology and mRNA platforms, as seen in the COVID-19 vaccines, allowing for quicker responses to emerging infectious diseases.
4. **How is personalized medicine advancing through biotechnology?**
Personalized medicine uses biotechnological tools to tailor medical treatment to individual characteristics, such as genetic makeup, improving the efficacy and safety of therapies for conditions like cancer and cardiovascular diseases.
5. **What are biosensors and their application in healthcare?**
Biosensors are analytical devices that convert a biological response into an electrical signal. In healthcare, they are used for monitoring glucose levels in diabetes patients, detecting pathogens, and measuring biomarkers for various diseases.
6. **How is biotechnology contributing to regenerative medicine?**
Biotechnology contributes to regenerative medicine by developing stem cell therapies and tissue engineering techniques, which aim to repair or replace damaged tissues and organs, offering potential treatments for conditions like heart disease and spinal cord injuries.Biotechnology has significantly advanced healthcare by revolutionizing the diagnosis, treatment, and prevention of diseases. Through genetic engineering, personalized medicine, and biopharmaceuticals, biotechnology has enabled more precise and effective interventions. Innovations such as CRISPR gene editing, monoclonal antibodies, and mRNA vaccines have transformed therapeutic approaches, offering new hope for previously untreatable conditions. Additionally, biotechnology has enhanced diagnostic capabilities with rapid and accurate tests, improving early detection and management of diseases. As research continues to progress, biotechnology holds the promise of further breakthroughs, potentially leading to cures for genetic disorders, more effective cancer treatments, and improved management of chronic diseases, ultimately contributing to longer, healthier lives.
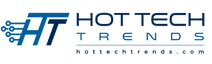