Unraveling the intricate tapestry of protein evolution offers profound insights into the origins of life on Earth. Proteins, the workhorses of cellular machinery, are fundamental to understanding how life has evolved from simple molecules to complex organisms. By tracing the evolutionary pathways of proteins, scientists can reconstruct the molecular history that underpins biological diversity and complexity. This exploration not only sheds light on the ancient biochemical processes that gave rise to life but also enhances our understanding of how proteins have adapted to various environmental challenges over billions of years. Through advanced techniques in molecular biology, bioinformatics, and evolutionary biology, researchers are piecing together the evolutionary narrative of proteins, providing a window into the primordial world and the evolutionary forces that have shaped life as we know it.
Exploring Ancestral Proteins: Insights Into Early Life Forms
The study of ancestral proteins offers a fascinating window into the early life forms that once inhabited our planet, providing crucial insights into the origins and evolution of life. By examining these ancient proteins, scientists can trace the evolutionary pathways that have led to the diversity of life we see today. This exploration not only enhances our understanding of biological history but also sheds light on the fundamental processes that have shaped life on Earth.
To begin with, proteins are essential molecules that perform a myriad of functions within living organisms. They are composed of amino acids, which are linked together in specific sequences dictated by genetic information. Over time, these sequences have undergone changes due to mutations, leading to the evolution of new proteins with novel functions. By reconstructing ancestral proteins, researchers can infer the characteristics of early life forms and the environments in which they thrived.
One of the primary methods used to study ancestral proteins is the reconstruction of ancient gene sequences. Scientists employ computational techniques to analyze the genetic information of modern organisms, identifying conserved sequences that have been preserved through evolutionary time. By comparing these sequences across different species, researchers can infer the genetic makeup of common ancestors and, consequently, the structure and function of their proteins. This approach allows scientists to recreate proteins that existed millions or even billions of years ago, providing a glimpse into the molecular machinery of early life.
Furthermore, the study of ancestral proteins has revealed significant insights into the adaptability and resilience of early life forms. For instance, by examining proteins from ancient organisms that lived in extreme environments, such as high temperatures or acidic conditions, researchers have gained a better understanding of how life can thrive under harsh conditions. These findings have implications not only for evolutionary biology but also for biotechnology, as they can inform the development of robust enzymes for industrial applications.
In addition to understanding the environmental adaptations of early life, ancestral protein studies have also illuminated the evolutionary innovations that have driven the complexity of life. For example, the evolution of proteins involved in photosynthesis marked a pivotal moment in Earth’s history, leading to the proliferation of oxygen and the rise of complex multicellular organisms. By reconstructing these ancient proteins, scientists can explore how such critical functions emerged and evolved over time.
Moreover, the exploration of ancestral proteins has provided valuable insights into the origins of diseases. Many modern diseases are linked to genetic mutations that affect protein function. By studying the evolutionary history of these proteins, researchers can identify the ancestral states that were more stable or functional, offering potential targets for therapeutic intervention. This evolutionary perspective can guide the development of treatments that restore or mimic the function of ancestral proteins, potentially alleviating the effects of genetic disorders.
In conclusion, the study of ancestral proteins is a powerful tool for unraveling the mysteries of early life forms and their evolutionary trajectories. By reconstructing these ancient molecules, scientists can gain a deeper understanding of the origins and evolution of life on Earth. This research not only enriches our knowledge of biological history but also holds promise for practical applications in medicine and biotechnology. As we continue to explore the molecular remnants of our distant past, we are reminded of the intricate tapestry of life that has unfolded over billions of years, shaping the world we inhabit today.
Molecular Archaeology: Reconstructing Ancient Protein Structures
In the quest to understand the origins of life, scientists have turned their attention to the molecular building blocks that underpin all living organisms: proteins. These complex molecules, composed of long chains of amino acids, perform a myriad of functions essential to life, from catalyzing biochemical reactions to providing structural support. The study of protein evolution, therefore, offers a window into the past, allowing researchers to trace the development of life on Earth. This field, often referred to as molecular archaeology, involves reconstructing ancient protein structures to gain insights into the early stages of biological evolution.
To begin with, proteins are encoded by genes, and as such, they are subject to the forces of evolution. Over millions of years, genetic mutations have introduced variations in protein sequences, leading to the diversity of life we observe today. By comparing the sequences of proteins from different organisms, scientists can infer the evolutionary relationships between species and identify common ancestors. This comparative approach has been instrumental in reconstructing the tree of life, providing a framework for understanding how complex life forms have evolved from simpler ones.
However, the task of reconstructing ancient protein structures is not without its challenges. The fossil record, while invaluable for studying the morphology of extinct organisms, offers little information about the molecular composition of ancient life. To overcome this limitation, researchers employ a technique known as ancestral sequence reconstruction. This method involves using computational algorithms to infer the sequences of ancient proteins based on the sequences of their modern descendants. By simulating the evolutionary process, scientists can predict the most likely sequences of proteins that existed in the distant past.
Once these ancient sequences are reconstructed, they can be synthesized in the laboratory and studied to understand their properties and functions. This experimental approach allows researchers to test hypotheses about the roles these proteins may have played in early life forms. For instance, by examining the stability and activity of reconstructed proteins under different environmental conditions, scientists can infer the types of environments in which ancient organisms might have thrived. Such insights are crucial for piecing together the puzzle of life’s origins, as they provide clues about the conditions that favored the emergence and evolution of life on Earth.
Moreover, the study of ancient proteins has implications beyond understanding the past. It can also inform our understanding of modern proteins and their functions. By examining how proteins have evolved over time, researchers can identify the key structural features that have been conserved throughout evolution. These conserved elements often play critical roles in protein function, and understanding them can aid in the design of new proteins with specific properties for applications in medicine and biotechnology.
In conclusion, molecular archaeology, through the reconstruction of ancient protein structures, offers a powerful tool for unraveling the mysteries of life’s origins. By bridging the gap between the past and present, this field not only enhances our understanding of evolutionary processes but also provides valuable insights that can drive future scientific and technological advancements. As researchers continue to refine their methods and expand their knowledge, the study of protein evolution promises to shed light on some of the most fundamental questions about the nature of life itself.
Evolutionary Pathways: How Proteins Adapted Over Millennia
The study of protein evolution offers a fascinating glimpse into the molecular underpinnings of life, tracing back to the very origins of biological complexity. Proteins, as the workhorses of the cell, play crucial roles in virtually every biological process, from catalyzing metabolic reactions to providing structural support. Understanding how proteins have adapted over millennia not only sheds light on the history of life on Earth but also provides insights into the mechanisms of evolution itself.
To begin with, proteins are composed of amino acids, which are linked together in specific sequences dictated by genetic information. The sequence of amino acids determines a protein’s three-dimensional structure and, consequently, its function. Over evolutionary time, these sequences have undergone changes through mutations, which can be neutral, deleterious, or occasionally beneficial. Beneficial mutations are preserved through natural selection, allowing organisms to adapt to their environments. This process of mutation and selection is central to the evolution of proteins.
One of the most compelling aspects of protein evolution is the concept of molecular convergence, where different proteins independently evolve similar structures or functions. This phenomenon highlights the constraints and opportunities presented by the biochemical landscape. For instance, enzymes that catalyze similar reactions in different species often exhibit convergent evolution, suggesting that there are optimal solutions to specific biochemical challenges. This convergence underscores the idea that while evolution is a random process, it is also shaped by the functional demands placed on proteins.
Furthermore, the study of ancient proteins, or paleoproteomics, has provided remarkable insights into the evolutionary pathways of proteins. By reconstructing ancestral proteins, scientists can infer the properties of proteins that existed millions of years ago. These reconstructions have revealed that early proteins were often more thermally stable, reflecting the hot environments of early Earth. As the planet cooled, proteins adapted to new environmental conditions, demonstrating the dynamic nature of protein evolution.
In addition to environmental pressures, gene duplication has played a significant role in protein evolution. When a gene is duplicated, it provides an opportunity for one copy to acquire new functions while the other maintains its original role. This process, known as neofunctionalization, has led to the diversification of protein functions and the emergence of new biological pathways. Gene duplication events are evident in the evolutionary history of many protein families, illustrating how genetic redundancy can drive innovation.
Moreover, horizontal gene transfer, particularly in prokaryotes, has facilitated the rapid spread of advantageous protein functions across different species. This mechanism allows organisms to acquire new capabilities without waiting for the slow process of mutation and selection. Horizontal gene transfer has been instrumental in the evolution of antibiotic resistance, highlighting its impact on protein evolution and adaptation.
In conclusion, the evolution of proteins is a complex interplay of genetic variation, environmental pressures, and molecular constraints. By unraveling the evolutionary pathways of proteins, scientists gain a deeper understanding of how life has adapted and thrived over billions of years. This knowledge not only enriches our understanding of biology but also informs fields such as medicine and biotechnology, where insights into protein function and evolution can lead to novel therapeutic strategies and innovations. As research in this area continues to advance, it promises to reveal even more about the intricate tapestry of life and the molecular evolution that underpins it.
The Role Of Mutations In Protein Evolution And Diversity
Proteins, the workhorses of the cell, are fundamental to the myriad processes that sustain life. Their evolution is a fascinating journey that traces back to the origins of life itself. Central to this evolutionary process is the role of mutations, which serve as the primary drivers of protein diversity. Understanding how mutations contribute to protein evolution provides insight into the mechanisms that have shaped the complexity of life on Earth.
Mutations, which are changes in the genetic sequence, can occur spontaneously or be induced by environmental factors. These alterations in DNA can lead to changes in the amino acid sequence of proteins, potentially affecting their structure and function. While many mutations are neutral or deleterious, some confer advantageous traits that can be subject to natural selection. Over time, beneficial mutations accumulate, leading to the evolution of new protein functions and, consequently, the diversification of life forms.
The process of mutation and selection is not random but rather a dynamic interplay that allows organisms to adapt to their environments. For instance, a single point mutation might alter an enzyme’s active site, enhancing its catalytic efficiency or allowing it to process a different substrate. Such changes can provide a selective advantage, enabling organisms to exploit new ecological niches. This adaptability is a testament to the power of mutations in driving evolutionary innovation.
Moreover, mutations can lead to the duplication of genes, a process that significantly contributes to protein diversity. Gene duplication allows one copy of a gene to maintain its original function while the other is free to accumulate mutations. This can result in the emergence of proteins with novel functions, a phenomenon known as neofunctionalization. The evolution of hemoglobin and myoglobin from a common ancestral gene is a classic example of how gene duplication and subsequent mutations have led to specialized proteins with distinct roles in oxygen transport and storage.
In addition to point mutations and gene duplications, other genetic mechanisms such as insertions, deletions, and recombination events also play crucial roles in protein evolution. These processes can create new protein domains or alter existing ones, further expanding the functional repertoire of proteins. The modular nature of protein domains allows for the shuffling and recombination of functional units, facilitating the evolution of complex protein architectures.
While mutations are the raw material for evolution, the environment acts as a selective pressure that shapes the trajectory of protein evolution. Changes in environmental conditions, such as temperature, pH, or the presence of toxins, can influence which mutations are advantageous. This interaction between genetic variation and environmental factors underscores the adaptive nature of protein evolution.
Furthermore, the study of protein evolution is not only of academic interest but also has practical implications. Understanding how proteins evolve can inform the development of new drugs and therapies, as well as the engineering of proteins with desired properties for industrial applications. By unraveling the molecular mechanisms underlying protein evolution, scientists can harness this knowledge to address pressing challenges in medicine, agriculture, and biotechnology.
In conclusion, mutations are pivotal in driving the evolution and diversity of proteins, shaping the complexity of life from its earliest origins. Through a combination of genetic variation and natural selection, proteins have evolved to perform an astonishing array of functions, enabling organisms to adapt and thrive in diverse environments. As research continues to uncover the intricacies of protein evolution, it promises to deepen our understanding of life’s evolutionary history and its future potential.
Comparative Genomics: Tracing Protein Lineages Across Species
In the quest to understand the origins of life, scientists have turned to the study of proteins, the complex molecules that perform a myriad of functions essential to life. Proteins are not only the workhorses of the cell but also serve as a historical record of evolutionary processes. By examining the sequences and structures of proteins across different species, researchers can trace the lineage of these molecules back to the earliest forms of life. This approach, known as comparative genomics, provides a window into the evolutionary history of proteins and, by extension, the organisms that harbor them.
Comparative genomics involves the analysis of genetic material from multiple species to identify similarities and differences in their protein-coding genes. This method allows scientists to infer the evolutionary relationships between species and to reconstruct the ancestral proteins that gave rise to modern variants. By comparing the genomes of diverse organisms, researchers can identify conserved protein sequences that have remained unchanged over millions of years, suggesting their critical role in fundamental biological processes. These conserved sequences, often referred to as “molecular fossils,” provide clues about the functions of ancient proteins and the environmental pressures that shaped their evolution.
One of the key insights gained from comparative genomics is the concept of homologous proteins, which are proteins that share a common evolutionary origin. Homologous proteins can be further classified into orthologs and paralogs. Orthologs are proteins in different species that evolved from a common ancestral gene and typically retain the same function. In contrast, paralogs arise from gene duplication events within a single species and may evolve new functions. By distinguishing between orthologs and paralogs, scientists can better understand the functional diversification of proteins and the evolutionary mechanisms that drive innovation in the protein repertoire of organisms.
The study of protein evolution through comparative genomics has also shed light on the phenomenon of convergent evolution, where unrelated species independently evolve similar protein structures or functions in response to similar environmental challenges. This process highlights the adaptive nature of protein evolution and underscores the importance of structural and functional constraints in shaping protein diversity. By identifying instances of convergent evolution, researchers can gain insights into the selective pressures that have influenced protein evolution and the strategies that organisms have employed to overcome similar challenges.
Furthermore, comparative genomics has revealed the role of horizontal gene transfer in protein evolution. This process involves the transfer of genetic material between unrelated species, leading to the acquisition of new proteins and functions. Horizontal gene transfer is particularly prevalent in prokaryotes, where it contributes to the rapid adaptation of bacteria to new environments and the spread of antibiotic resistance. By tracing the origins of horizontally transferred proteins, scientists can better understand the dynamics of microbial evolution and the impact of gene exchange on the evolution of complex traits.
In conclusion, comparative genomics provides a powerful framework for unraveling the evolutionary history of proteins and tracing their lineage across species. By examining the genetic blueprints of diverse organisms, researchers can reconstruct the ancestral proteins that laid the foundation for life as we know it. This approach not only enhances our understanding of protein evolution but also offers valuable insights into the broader processes that have shaped the diversity of life on Earth. As genomic technologies continue to advance, the study of protein evolution will undoubtedly yield further discoveries, deepening our understanding of life’s origins and the intricate web of evolutionary relationships that connect all living organisms.
The Impact Of Environmental Changes On Protein Evolution
The study of protein evolution offers profound insights into the origins of life, revealing how environmental changes have shaped the molecular machinery that underpins all living organisms. Proteins, as the workhorses of the cell, perform a myriad of functions essential for life, from catalyzing biochemical reactions to providing structural support. Understanding how proteins have evolved in response to environmental shifts is crucial for unraveling the complex history of life on Earth.
Environmental changes, whether gradual or abrupt, have exerted significant pressure on the evolutionary trajectory of proteins. These changes can include fluctuations in temperature, pH levels, salinity, and the availability of nutrients, each of which can influence protein stability and functionality. For instance, the transition from an anoxic to an oxygen-rich atmosphere approximately 2.4 billion years ago, known as the Great Oxidation Event, necessitated the evolution of proteins capable of coping with oxidative stress. This event led to the emergence of new protein families, such as those involved in antioxidant defense mechanisms, which were crucial for the survival of early aerobic organisms.
Moreover, the adaptation of proteins to extreme environments, such as hydrothermal vents or polar ice caps, provides further evidence of the impact of environmental changes on protein evolution. Proteins in extremophiles, organisms that thrive in extreme conditions, have evolved unique structural features that confer stability and functionality under such harsh conditions. For example, proteins from thermophilic organisms, which live in high-temperature environments, often exhibit increased numbers of ionic bonds and hydrophobic interactions, enhancing their thermal stability. These adaptations highlight the remarkable plasticity of proteins and their ability to evolve in response to environmental pressures.
In addition to physical and chemical changes, biological factors such as the emergence of new species and ecosystems have also influenced protein evolution. The Cambrian Explosion, a period of rapid diversification of life forms approximately 540 million years ago, saw the development of novel protein structures and functions. This diversification was driven by the need for organisms to adapt to new ecological niches and interactions, leading to the evolution of proteins involved in processes such as cell signaling, immune response, and multicellularity.
Furthermore, the study of ancient proteins, or paleoproteomics, has provided valuable insights into how proteins have evolved over geological timescales. By reconstructing ancestral protein sequences and comparing them with modern counterparts, researchers can infer the evolutionary pressures that have shaped protein function and stability. This approach has revealed that many proteins have undergone significant changes in response to environmental shifts, underscoring the dynamic nature of protein evolution.
In conclusion, the impact of environmental changes on protein evolution is a testament to the adaptability and resilience of life. As proteins continue to evolve in response to ongoing environmental changes, such as climate change and pollution, understanding their evolutionary history becomes increasingly important. This knowledge not only sheds light on the origins of life but also informs efforts to engineer proteins with novel functions for biotechnological applications. By tracing the evolutionary pathways of proteins, scientists can better appreciate the intricate interplay between life and its ever-changing environment, offering a window into the past and a guide for the future.
Q&A
1. **What is protein evolution?**
Protein evolution refers to the process by which protein structures and functions change over time through genetic mutations, natural selection, and other evolutionary mechanisms.
2. **How do scientists trace protein evolution back to life’s origins?**
Scientists trace protein evolution by comparing protein sequences across different species, using bioinformatics tools to reconstruct ancestral proteins, and studying the molecular mechanisms that drive evolutionary changes.
3. **What role do mutations play in protein evolution?**
Mutations introduce changes in the amino acid sequences of proteins, which can alter their structure and function. Some mutations are beneficial and become fixed in a population, contributing to evolutionary adaptation.
4. **Why is understanding protein evolution important for understanding life’s origins?**
Understanding protein evolution helps scientists uncover how early proteins might have functioned, providing insights into the biochemical processes that were crucial for the emergence and diversification of life.
5. **What are some methods used to study ancient proteins?**
Methods include comparative genomics, phylogenetic analysis, and experimental techniques like ancestral protein reconstruction and laboratory evolution to study the properties of ancient proteins.
6. **How does protein evolution contribute to biodiversity?**
Protein evolution leads to the development of new protein functions and adaptations, which can result in the emergence of new species and contribute to the vast diversity of life forms on Earth.Unraveling protein evolution provides critical insights into the origins of life by tracing the molecular changes that have occurred over billions of years. By examining the structure, function, and genetic sequences of proteins across diverse organisms, scientists can reconstruct evolutionary pathways and identify ancestral proteins that likely existed in early life forms. This research not only enhances our understanding of how complex biological systems have evolved but also sheds light on the fundamental processes that have driven the diversification of life on Earth. Ultimately, studying protein evolution helps bridge the gap between the molecular mechanisms of ancient life and the vast biodiversity we observe today, offering a deeper appreciation of the interconnectedness of all living organisms.
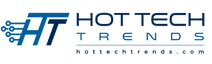