Pioneering breakthroughs in drug and medicine development have revolutionized the landscape of healthcare, offering unprecedented opportunities to combat diseases and improve patient outcomes. These advancements are the result of relentless research, cutting-edge technology, and innovative methodologies that have transformed the way we understand and treat various medical conditions. From the discovery of novel therapeutic targets to the development of personalized medicine, the field has witnessed remarkable progress. Breakthroughs such as gene editing, immunotherapy, and artificial intelligence-driven drug discovery are not only enhancing the efficacy and safety of treatments but also accelerating the pace at which new therapies reach the market. As we continue to push the boundaries of science and technology, these pioneering efforts hold the promise of addressing some of the most pressing health challenges of our time, ultimately paving the way for a healthier future.
Gene Editing Technologies: Revolutionizing Precision Medicine
Gene editing technologies have emerged as a transformative force in the field of precision medicine, offering unprecedented opportunities to tailor medical treatments to individual genetic profiles. At the forefront of these technologies is CRISPR-Cas9, a revolutionary tool that allows scientists to make precise modifications to the DNA of living organisms. This innovation has opened new avenues for understanding and treating a myriad of genetic disorders, thereby revolutionizing the landscape of drug and medicine development.
The precision of CRISPR-Cas9 lies in its ability to target specific sequences of DNA, enabling researchers to edit genes with remarkable accuracy. This capability is particularly significant in the context of precision medicine, where the goal is to develop treatments that are specifically designed to address the unique genetic makeup of each patient. By correcting genetic mutations at their source, CRISPR-Cas9 holds the potential to cure diseases that were previously deemed incurable, such as cystic fibrosis, sickle cell anemia, and certain forms of cancer.
Moreover, the application of gene editing technologies extends beyond the treatment of genetic disorders. In the realm of oncology, for instance, CRISPR-Cas9 is being utilized to enhance the efficacy of immunotherapies. By editing the genes of immune cells, scientists can bolster their ability to recognize and attack cancer cells, thereby improving patient outcomes. This approach exemplifies the broader trend of leveraging gene editing to refine and optimize existing therapeutic strategies, ultimately leading to more effective and personalized treatments.
In addition to CRISPR-Cas9, other gene editing technologies, such as TALENs and zinc finger nucleases, continue to play a crucial role in advancing precision medicine. While these tools may not offer the same level of simplicity and efficiency as CRISPR-Cas9, they provide alternative methods for gene editing that can be tailored to specific applications. This diversity of tools underscores the dynamic nature of the field and highlights the ongoing efforts to refine and expand the capabilities of gene editing technologies.
Despite the promising potential of gene editing, ethical considerations remain a significant concern. The ability to alter the human genome raises profound questions about the implications of such interventions, particularly in the context of germline editing, where changes can be passed on to future generations. As the technology continues to advance, it is imperative that researchers, ethicists, and policymakers engage in ongoing dialogue to establish guidelines that ensure the responsible and ethical use of gene editing.
Furthermore, the accessibility and affordability of gene editing technologies are critical factors that will determine their impact on global health. Efforts to democratize access to these tools are essential to ensure that the benefits of precision medicine are equitably distributed across diverse populations. This includes addressing disparities in healthcare infrastructure and investing in education and training to build the necessary expertise in regions that are currently underserved.
In conclusion, gene editing technologies represent a pivotal advancement in the pursuit of precision medicine, offering the potential to transform the way we understand and treat diseases. As research continues to evolve, the integration of these technologies into clinical practice will require careful consideration of ethical, social, and economic factors. By navigating these challenges thoughtfully, the medical community can harness the full potential of gene editing to improve health outcomes and usher in a new era of personalized medicine.
AI and Machine Learning in Drug Discovery
The integration of artificial intelligence (AI) and machine learning (ML) into drug discovery represents a transformative shift in the pharmaceutical industry, promising to revolutionize the way new medicines are developed. Traditionally, drug discovery has been a time-consuming and costly process, often taking over a decade and billions of dollars to bring a new drug to market. However, the advent of AI and ML technologies is poised to significantly reduce these barriers, offering unprecedented opportunities for innovation and efficiency.
AI and ML algorithms excel at analyzing vast datasets, identifying patterns, and making predictions, capabilities that are particularly valuable in the complex field of drug discovery. By leveraging these technologies, researchers can sift through enormous amounts of biological data to identify potential drug candidates more quickly and accurately than ever before. For instance, AI can analyze genomic data to identify new drug targets, predict how different compounds will interact with these targets, and even suggest modifications to improve efficacy and reduce side effects. This ability to rapidly process and interpret data not only accelerates the initial stages of drug discovery but also enhances the precision of the entire process.
Moreover, AI and ML are instrumental in optimizing the design of clinical trials, which are critical yet resource-intensive components of drug development. By using predictive analytics, AI can help identify the most suitable patient populations for specific trials, thereby increasing the likelihood of success and reducing the time required to obtain conclusive results. Additionally, machine learning models can continuously analyze data from ongoing trials, providing real-time insights that can inform adjustments to trial protocols, ultimately leading to more efficient and effective studies.
Furthermore, the application of AI in drug repurposing is another promising area. Drug repurposing involves finding new therapeutic uses for existing drugs, a process that can be significantly expedited by AI’s ability to analyze existing drug data and identify potential new applications. This approach not only reduces the time and cost associated with developing new drugs from scratch but also leverages the safety profiles of existing drugs, thereby minimizing the risks associated with new drug development.
Despite these promising advancements, the integration of AI and ML in drug discovery is not without challenges. One of the primary concerns is the quality and reliability of the data used to train AI models. Inaccurate or biased data can lead to erroneous predictions, potentially compromising the safety and efficacy of new drugs. Therefore, ensuring the integrity of data and developing robust validation methods are critical to the successful application of AI in this field.
Additionally, the regulatory landscape for AI-driven drug discovery is still evolving. Regulatory agencies must adapt to the rapid pace of technological advancement, developing frameworks that ensure the safety and efficacy of AI-developed drugs while fostering innovation. Collaboration between industry stakeholders, regulatory bodies, and technology developers will be essential to navigate these challenges and fully realize the potential of AI in drug discovery.
In conclusion, the incorporation of AI and ML into drug discovery is a pioneering breakthrough that holds the promise of transforming the pharmaceutical industry. By enhancing the speed, accuracy, and efficiency of drug development, these technologies have the potential to bring new, life-saving medicines to patients more quickly and at a lower cost. As the field continues to evolve, ongoing collaboration and innovation will be key to overcoming challenges and unlocking the full potential of AI in drug discovery.
Personalized Medicine: Tailoring Treatments to Individual Genomes
In recent years, the field of medicine has witnessed a transformative shift towards personalized medicine, a groundbreaking approach that tailors medical treatment to the individual characteristics of each patient. This paradigm shift is largely driven by advancements in genomic research, which have unveiled the intricate details of human DNA and its role in health and disease. Personalized medicine, also known as precision medicine, leverages this genomic information to develop targeted therapies that are more effective and have fewer side effects compared to traditional one-size-fits-all treatments.
The foundation of personalized medicine lies in the understanding that each individual’s genetic makeup is unique, influencing their susceptibility to certain diseases and their response to various treatments. By analyzing a patient’s genome, healthcare providers can identify specific genetic variations that may contribute to the development of diseases such as cancer, cardiovascular disorders, and rare genetic conditions. This genetic insight allows for the customization of treatment plans that are specifically designed to target the underlying genetic causes of a patient’s condition.
One of the most significant breakthroughs in personalized medicine is the development of targeted therapies for cancer treatment. Traditional chemotherapy, while effective in killing cancer cells, often affects healthy cells as well, leading to severe side effects. In contrast, targeted therapies are designed to attack specific genetic mutations found in cancer cells, sparing healthy cells and reducing adverse effects. For instance, the drug imatinib has revolutionized the treatment of chronic myeloid leukemia by specifically targeting the BCR-ABL fusion protein, a genetic abnormality present in most patients with this type of cancer. This targeted approach has significantly improved survival rates and quality of life for patients.
Moreover, personalized medicine extends beyond cancer treatment. In the realm of cardiovascular diseases, genetic testing can identify individuals at high risk for conditions such as familial hypercholesterolemia, a genetic disorder characterized by high cholesterol levels. Early identification allows for proactive management through lifestyle changes and tailored medication regimens, potentially preventing the onset of heart disease. Similarly, in the field of pharmacogenomics, understanding how genetic variations affect drug metabolism enables healthcare providers to prescribe medications that are most effective for each patient, minimizing the risk of adverse drug reactions.
The integration of personalized medicine into clinical practice is not without challenges. The complexity of the human genome and the vast amount of data generated by genomic sequencing require sophisticated analytical tools and expertise. Additionally, ethical considerations surrounding genetic privacy and data security must be addressed to ensure patient trust and confidentiality. Despite these challenges, the potential benefits of personalized medicine are immense, promising a future where treatments are not only more effective but also more aligned with the individual needs of patients.
In conclusion, personalized medicine represents a pioneering breakthrough in drug and medicine development, offering a more precise and individualized approach to healthcare. By harnessing the power of genomics, this innovative field is transforming the way diseases are diagnosed, treated, and prevented. As research continues to advance, personalized medicine holds the promise of improving patient outcomes and revolutionizing the landscape of modern medicine, ultimately leading to a new era of healthcare that is as unique as the individuals it serves.
Nanotechnology in Drug Delivery Systems
Nanotechnology has emerged as a transformative force in the field of drug delivery systems, offering unprecedented opportunities to enhance the efficacy and precision of medical treatments. This innovative approach leverages the unique properties of materials at the nanoscale, typically ranging from 1 to 100 nanometers, to address some of the most pressing challenges in medicine. As researchers continue to explore the potential of nanotechnology, it is becoming increasingly clear that this field holds the key to pioneering breakthroughs in drug and medicine development.
One of the most significant advantages of nanotechnology in drug delivery is its ability to improve the bioavailability of therapeutic agents. Many drugs, particularly those that are poorly soluble in water, face significant obstacles in reaching their target sites within the body. Nanoparticles can be engineered to encapsulate these drugs, enhancing their solubility and stability. This not only ensures that a higher concentration of the drug reaches the intended site but also reduces the frequency and dosage required, thereby minimizing potential side effects.
Moreover, nanotechnology enables targeted drug delivery, a crucial advancement in the treatment of diseases such as cancer. Traditional chemotherapy, for instance, affects both cancerous and healthy cells, leading to severe side effects. In contrast, nanoparticles can be designed to recognize and bind to specific markers on cancer cells, delivering the drug directly to the tumor while sparing healthy tissue. This targeted approach not only increases the therapeutic efficacy but also significantly reduces the adverse effects associated with conventional treatments.
In addition to improving drug delivery, nanotechnology also facilitates the development of novel therapeutic agents. For example, researchers are exploring the use of nanoscale materials such as liposomes, dendrimers, and quantum dots to create new drug formulations with enhanced properties. These materials can be engineered to release drugs in a controlled manner, responding to specific stimuli such as pH changes or temperature variations within the body. This level of control allows for more precise treatment regimens, tailored to the individual needs of patients.
Furthermore, the integration of nanotechnology with other cutting-edge fields, such as genomics and personalized medicine, is paving the way for even more groundbreaking advancements. By combining the precision of nanotechnology with the insights gained from genetic profiling, it is possible to develop highly personalized treatment plans that take into account a patient’s unique genetic makeup. This approach not only maximizes the effectiveness of the treatment but also minimizes the risk of adverse reactions, leading to better patient outcomes.
Despite the promising potential of nanotechnology in drug delivery systems, there are still challenges that need to be addressed. The long-term safety and biocompatibility of nanoparticles remain a concern, necessitating rigorous testing and evaluation. Additionally, the complexity of manufacturing and scaling up these technologies poses significant hurdles that must be overcome to ensure their widespread adoption.
In conclusion, nanotechnology is revolutionizing the field of drug delivery systems, offering innovative solutions to longstanding challenges in medicine. By enhancing drug bioavailability, enabling targeted delivery, and facilitating the development of novel therapeutic agents, this technology holds the promise of significantly improving patient care. As research continues to advance, it is likely that nanotechnology will play an increasingly central role in the development of new and more effective medical treatments, heralding a new era in healthcare.
The Role of Biologics in Modern Therapeutics
In recent years, the field of drug and medicine development has witnessed remarkable advancements, particularly with the emergence and growing prominence of biologics. These complex molecules, derived from living cells, have revolutionized the landscape of modern therapeutics, offering new avenues for treatment that were previously unimaginable. As we delve into the role of biologics in contemporary medicine, it becomes evident that their impact is profound and multifaceted, reshaping how we approach the treatment of various diseases.
Biologics, which include a wide range of products such as monoclonal antibodies, vaccines, and cell therapies, are distinguished by their ability to target specific components of the immune system or disease pathways with high precision. This specificity is a significant advantage over traditional small-molecule drugs, which often affect multiple pathways and can lead to unintended side effects. Consequently, biologics have opened new doors in the treatment of complex diseases, including cancer, autoimmune disorders, and rare genetic conditions, where traditional therapies have fallen short.
One of the most notable contributions of biologics is in the realm of oncology. Monoclonal antibodies, for instance, have been engineered to recognize and bind to specific antigens on cancer cells, marking them for destruction by the immune system. This targeted approach not only enhances the efficacy of treatment but also minimizes damage to healthy cells, thereby reducing the adverse effects commonly associated with chemotherapy. Furthermore, the advent of immune checkpoint inhibitors, a class of biologics, has transformed cancer therapy by unleashing the body’s immune system to attack tumors more effectively.
In addition to cancer, biologics have made significant strides in the management of autoimmune diseases such as rheumatoid arthritis, psoriasis, and inflammatory bowel disease. These conditions, characterized by an overactive immune response, have traditionally been challenging to treat. However, biologics that inhibit specific cytokines or immune cells have provided patients with more effective and sustainable treatment options, improving their quality of life and reducing disease progression.
Moreover, the development of biologics has been instrumental in addressing rare genetic disorders. Enzyme replacement therapies, a subset of biologics, have been developed to compensate for deficient or malfunctioning enzymes in patients with conditions like Gaucher’s disease and Fabry disease. These therapies have not only extended the lifespan of affected individuals but have also significantly improved their overall health outcomes.
Despite their transformative potential, the development and production of biologics present unique challenges. The complexity of these molecules requires sophisticated manufacturing processes and stringent quality control measures to ensure their safety and efficacy. Additionally, the high cost of biologics remains a barrier to accessibility for many patients. However, ongoing research and innovation in bioprocessing technologies are gradually addressing these challenges, paving the way for more cost-effective and widely available biologic therapies.
In conclusion, biologics have undeniably become a cornerstone of modern therapeutics, offering unprecedented opportunities for the treatment of a wide array of diseases. Their ability to provide targeted, effective, and personalized treatment options marks a significant departure from traditional drug development paradigms. As research continues to advance, the role of biologics is expected to expand further, heralding a new era in medicine where precision and efficacy are paramount. The ongoing exploration and refinement of these therapies hold promise for even greater breakthroughs in the future, ultimately improving patient outcomes and transforming healthcare as we know it.
CRISPR and Its Impact on Genetic Disorders
The advent of CRISPR technology has marked a revolutionary era in the field of genetic engineering, offering unprecedented possibilities for the treatment of genetic disorders. CRISPR, an acronym for Clustered Regularly Interspaced Short Palindromic Repeats, is a sophisticated tool that allows scientists to edit genes with remarkable precision. This technology has rapidly become a cornerstone in the development of new therapeutic strategies, fundamentally altering the landscape of medicine and drug development.
Initially discovered as a part of the immune system in bacteria, CRISPR has been adapted for use in human cells, enabling researchers to target and modify specific genetic sequences. This capability is particularly significant for genetic disorders, which are often caused by mutations in a single gene. By correcting these mutations, CRISPR holds the potential to not only treat but possibly cure a wide array of genetic conditions. For instance, diseases such as cystic fibrosis, sickle cell anemia, and muscular dystrophy, which have long posed challenges to traditional therapeutic approaches, are now being re-evaluated through the lens of CRISPR technology.
Moreover, the impact of CRISPR extends beyond the direct treatment of genetic disorders. It also plays a crucial role in drug development by facilitating the creation of more accurate disease models. These models are essential for understanding the pathophysiology of diseases and for testing the efficacy and safety of new drugs. By enabling precise genetic modifications, CRISPR allows for the development of cell and animal models that closely mimic human diseases, thereby enhancing the predictive power of preclinical studies.
In addition to its applications in disease modeling, CRISPR is instrumental in the identification of new drug targets. By systematically knocking out genes in cell lines, researchers can determine which genes are essential for the survival of diseased cells. This process, known as functional genomics, helps in pinpointing potential targets for therapeutic intervention. Consequently, CRISPR not only accelerates the drug discovery process but also increases the likelihood of developing effective treatments.
Despite its transformative potential, the use of CRISPR in medicine is not without challenges. Ethical considerations, particularly those related to germline editing, have sparked significant debate. The possibility of making heritable changes to the human genome raises profound ethical questions about the long-term implications for future generations. Furthermore, concerns about off-target effects, where unintended genetic modifications occur, underscore the need for rigorous safety assessments before CRISPR-based therapies can be widely adopted.
Nevertheless, ongoing research and technological advancements continue to address these challenges, paving the way for safer and more effective applications of CRISPR. As scientists refine the technology, the prospect of personalized medicine becomes increasingly attainable. By tailoring treatments to an individual’s genetic makeup, CRISPR has the potential to revolutionize healthcare, offering hope to patients with previously untreatable genetic disorders.
In conclusion, CRISPR technology represents a pioneering breakthrough in drug and medicine development, with profound implications for the treatment of genetic disorders. Its ability to precisely edit genes opens new avenues for therapeutic intervention, disease modeling, and drug discovery. While ethical and safety concerns remain, the continued evolution of CRISPR promises to transform the future of medicine, bringing us closer to a new era of personalized healthcare.
Q&A
1. **What was the first antibiotic discovered, and who discovered it?**
– Penicillin was the first antibiotic discovered by Alexander Fleming in 1928.
2. **What is CRISPR-Cas9, and why is it significant in medicine?**
– CRISPR-Cas9 is a gene-editing technology that allows for precise modifications to DNA. It is significant because it has the potential to correct genetic defects, treat diseases, and improve crop resilience.
3. **What breakthrough drug was developed for the treatment of HIV/AIDS in the 1990s?**
– Highly Active Antiretroviral Therapy (HAART) was developed in the 1990s, significantly improving the life expectancy and quality of life for people with HIV/AIDS.
4. **What is the significance of monoclonal antibodies in cancer treatment?**
– Monoclonal antibodies are significant because they can specifically target cancer cells, minimizing damage to healthy cells and improving the effectiveness of cancer treatments.
5. **What was the first vaccine developed using recombinant DNA technology?**
– The first vaccine developed using recombinant DNA technology was the hepatitis B vaccine, approved in 1986.
6. **What is the role of mRNA technology in recent vaccine development?**
– mRNA technology plays a crucial role in recent vaccine development, as seen with the COVID-19 vaccines, by enabling rapid and effective immune responses without using live virus components.Pioneering breakthroughs in drug and medicine development have revolutionized healthcare by introducing innovative therapies and treatments that address previously unmet medical needs. Advances in biotechnology, genomics, and personalized medicine have enabled the creation of targeted therapies that improve efficacy and reduce side effects. The integration of artificial intelligence and machine learning in drug discovery processes has accelerated the identification of potential drug candidates, significantly reducing the time and cost associated with bringing new drugs to market. Furthermore, the development of novel drug delivery systems and regenerative medicine techniques has expanded the possibilities for treating complex diseases. These breakthroughs not only enhance patient outcomes but also pave the way for a more precise and individualized approach to healthcare, ultimately transforming the landscape of modern medicine.
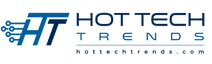